Various attempts to reduce the volume of greenhouse gas emissions are underway. One such effort that has long been the focus of attention is biomanufacturing, which leverages biotechnology and uses bioresources as basic ingredients, rather than relying on fossil resources. However, finding microorganisms that are suitable for use is difficult and the path to commercialization has been a long one. Now, though, cutting-edge technologies in the form of AI and genome editing are reducing that distance. Scientists say that it is possible to efficiently produce various synthetic chemical compounds without fossil resources by using genome editing technology to modify microorganisms according to designs created by AI.
Special Feature 1 – The Truth about Genome Editing “Manufacturing” bioresources using AI and genome-edited microorganisms
composition by Yuko Watanabe
Humanity is being forced to confront numerous issues in exchange for economic growth. Environmental pollution, climate change, food shortages, water shortages, population growth, resource depletion…all these global issues require us to take some kind of action without delay.
Biomass —— bioresources stemming from plants and animals
The concept of the circular economy lays the foundation for solutions to global issues. This is a socioeconomic system that aims to maximize added value, while making efficient, circular use of resources at every stage. As biotechnology researchers, we are endeavoring to bring this circular economy to fruition through advances in biomanufacturing, which involves leveraging genome editing and other cutting-edge biotechnologies to bring out the full capabilities of microorganisms and other biological organisms in order to produce various substances.
In the case of raw materials, for example, scientists believe that it will become possible to achieve the ultimate in material production by tapping into the power of biotechnology to create materials not only from renewable organic resources derived from organisms, but even, in the future, from CO2. This will free us from our reliance on petroleum and other fossil resources. Research and development efforts are progressing around the world, and competition is intensifying.
Carried out since ancient times, biomanufacturing is firmly established in our day-to-day lives. Foods such as miso, soy sauce, saké, pickles, natto, and yogurt have been transformed into new substances by means of a decomposition and fermentation process in which yeasts, Aspergillus oryzae, Lactic acid bacteria, and other such microorganisms use the sugars in the basic ingredients as a source of nutrition and metabolize them.
The basis of modern state-of-the-art biomanufacturing is the biorefinery, an environmentally friendly technology that produces alternatives to petroleum-based fuels and petrochemical products. Instead of fossil and other depletable resources, these biorefineries use biomass as their feedstocks. A form of bioresource stemming from plants and animals, which are sustainable organisms, biomass includes non-edible resource crops that do not compete with food sources, unused biomass such as agricultural and forest residues, waste paper, livestock feces, and sewage sludge.
Working on biorefinery research and development, our research team has been involved in constructing a value chain spanning the pretreatment of biomass, search for outstanding microorganisms, breeding of microorganisms, fermentation (material production), and separation and recovery of useful substances.
In the process of these research and development efforts, we focus in particular on searching for outstanding microorganisms and breeding them in order to enhance their innate capabilities (Figure 1). There are various different species of microorganisms, including the yeasts, Aspergillus oryzae, Lactic acid bacteria, Escherichia coli (E. coli), Actinomyces, and microalgae used in industry for the production of foods, chemicals, pharmaceuticals, cosmetics, and the like. However, it is not the case that any kind of microorganism is suitable for use in biomanufacturing. Development is not easy, because one has to identify which microorganisms have excellent properties and find out what kind of gene recombination or genome editing needs to be carried out to facilitate the breeding of high-performance microorganisms. Even if one discovers an outstanding microorganism and succeeds in developing it, one then needs to work out the culture method and conditions required to culture it on a commercial basis.
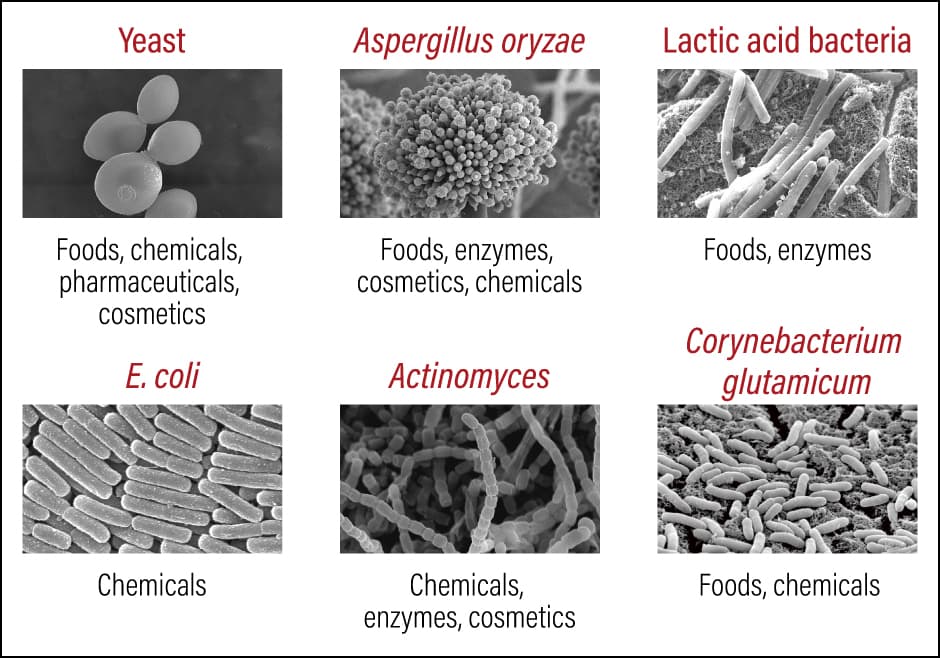
Figure 1. Fields of use of microorganismsScientists hope that, with the aid of biotechnology, microorganisms will become a key player in the circular economy by playing an active role in biomanufacturing.
High-performance microorganisms suited to mass production
However, we have now reached an age in which we can integrate the scientific knowledge we have amassed in such fields as biology and biochemistry with AI, robotics, and other technologies that continue to see remarkable advances, and can apply them to the search for microorganisms and their development. In addition, we are now able to substantially reduce the time required for microorganism development, which previously had to be carried out manually over a period of many months or even years. Next-generation sequencers can decode genomic information swiftly and within a short period, enabling us to modify genes with pinpoint accuracy by means of efficient, precise gene manipulation aided by genome editing.
The DBTL cycle is crucial to searching for and developing outstanding microorganisms. In the DBTL cycle, we use AI to design (D) a plan for modifying a microorganism’s metabolic pathways to equip it with a genome or gene suited to producing the target substance, and then use robots to build (B) diverse strains of microorganisms. After that, robots gather experimental data, which we process, including carrying out tests (T) to assess whether or not the design is correct. Finally, we use AI to learn (L) what improvements can be made, and then use this knowledge to produce the substance based on an even better design. This overall workflow is called the DBTL cycle. The new microorganisms generated from this cycle that are highly functional and capable of being mass-produced are called “smart cells.” The platform developed for the efficient production of smart cells based on the DBTL cycle is called a biofoundry, a name based on the foundries at which semiconductors are manufactured.
With smart cell development taking place across the globe, biofoundries are springing up to serve as research and development bases. As smart cell development has been positioned as part of a national strategy in Japan, Kobe University’s Engineering Biology Research Center opened Asia’s first biofoundry laboratory on Port Island in Kobe City in 2020, with support from the New Energy and Industrial Technology Development Organization (NEDO) (Figure 2). Smart cell development had taken several years using conventional techniques, but drawing on the technology we have developed will enable us to shorten the time required to less than a fifth. Accordingly, we aim to undertake joint development with a range of companies in an effort to create a smart cell industry, which will be a next-generation industry focused on the efficient production of high-performance chemicals, pharmaceuticals, and the like.
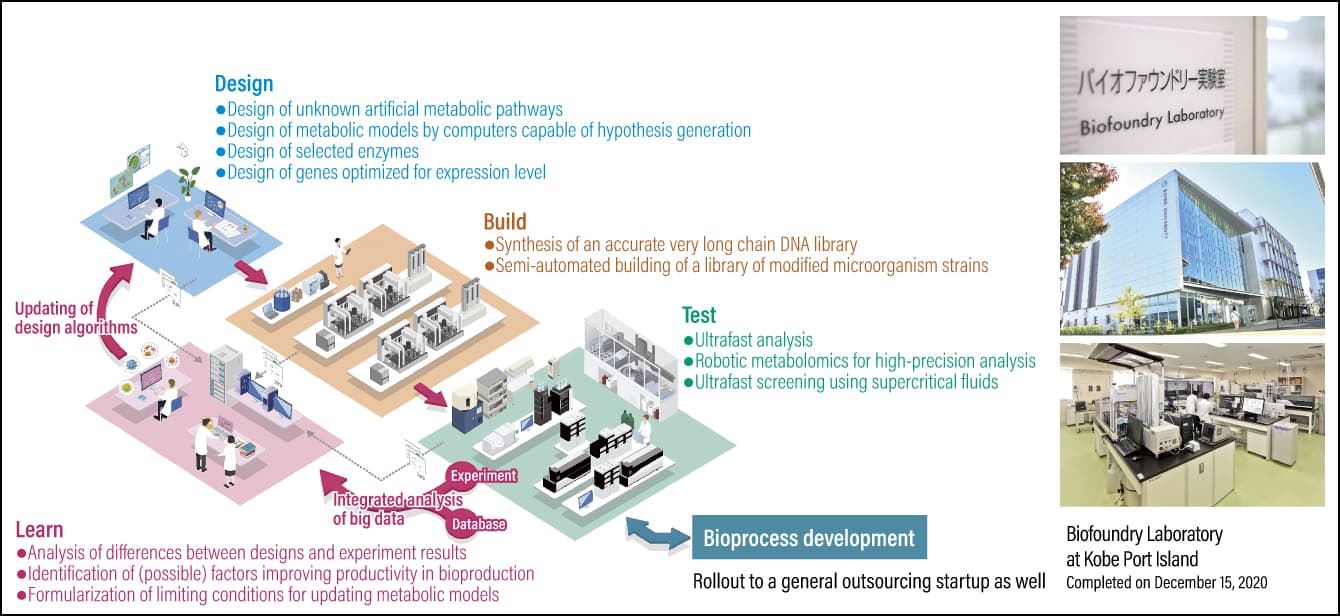
Figure 2. Smart cell creation platformKobe University’s Engineering Biology Research Center opened its Biofoundry Laboratory on Port Island in Kobe City in 2020, with support from NEDO. As Asia’s first smart cell creation platform based on the DBTL cycle, it aims to develop outstanding microorganisms in the form of smart cells.
At our university, we also partnered with Shimadzu Corporation in Kyoto City to develop Autonomous Lab, an autonomous experimental system that connects AI, robots, and the cloud to biomanufacturing. Over a period of three years, we have been verifying the usefulness of the prototype. We plan to progressively move forward with experiments that will enable robotic arms to transport samples to various modules, so that the necessary experiments can be carried out in the requisite place. The cloud system has been set up so as to enable large volumes of data to be uploaded automatically and checked by researchers, no matter where they might be. In addition, AI evaluates the results of the experiments and designs the next experiments that should be carried out; all humans have to do is check the designs, with the robots repeatedly conducting experiments.
As the ability to modify microorganisms in accordance with the AI-devised designs is absolutely crucial in biomanufacturing, genome editing technology is an essential tool. We make use of genome editing technology in a diverse array of experiments; when the automation of experimental procedures progresses, our research will undoubtedly advance even further. Laboratories across the globe are working hard on this area, and it is thought likely to become reality in another year or two.
Attention is also focusing on multiplex genome engineering, which enables multiple genes to be manipulated in a single procedure. This single gene manipulation procedure enables the expression levels of multiple genes to be raised or lowered. We believe that, if combined with robotics, it will enable us to try out a variety of combinations in a single experiment, thereby making it possible to learn the optimal combination within a short time.
With the emergence of this kind of system, people in all kinds of fields who are not experts in bioscience will be able to conduct experiments; we hope that this will help the biomanufacturing industry as a whole to flourish, and enable Japan to take the lead in intense international competition.
High hopes for microalgae and yeasts
So far, I have principally discussed the development of technologies and systems connected with biomanufacturing, but now I would like to introduce some actual results from the biomanufacturing research conducted by our team. Among the microorganisms that are key players in biomanufacturing, microalgae and yeasts show great promise as we continue our research (Figure 3).
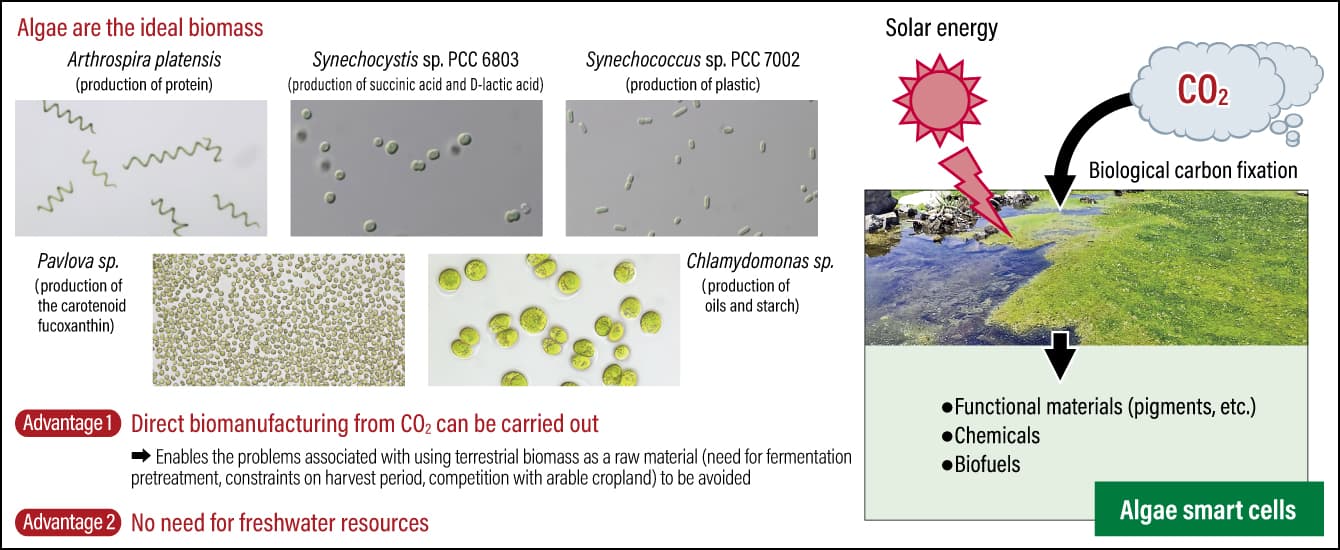
Figure 3. The future stars of biomanufacturingCyanobacteria —— a type of microalgae —— have come under the spotlight as the ultimate material-producing microorganism, because they can be used as a raw material in making biodegradable plastics. With the aid of genome editing technology, the research team led by Professor Hasunuma is conducting research aimed at increasing the potential for cyanobacteria to be used in bioproduction.
With regard to the first, microalgae are known to produce a variety of useful substances, including oils and pigments, from sunlight and CO2 alone. Formerly known as blue-green algae, cyanobacteria are a commonly used photosynthetic microorganism, because they have strong proliferation abilities and their genes can easily be modified. Cyanobacteria convert CO2 into a type of sugar called glycogen by means of photosynthesis, and store the glycogen in their cells. When transferred to a dark environment without oxygen, they metabolize the glycogen and release succinic acid, D-lactic acid , and other organic acids into the culture medium.
The metabolite D-lactic acid is the raw material used to make the bioplastic stereocomplex polylactic acid, which is a biodegradable plastic that breaks down completely to the molecular level and is ultimately circulated back into the natural world in the form of water and CO2. There is a technique for producing D-lactic acid that employs E. coli and other microorganisms in producing the material. However, as sugars (glucose) from maize, sugarcane, and the like are used as raw materials in a production process based on fermentation, this technique competes with food production for such resources as arable land and fresh water. Cyanobacteria, on the other hand, do not need to be cultured on land; as seawater can be used for many species and a variety of useful substances can be produced, hopes are high that they could be the ultimate material-producing microorganisms.
By means of dynamic metabolomics, our research team has shed light on the mechanism by which cyanobacteria produce D-lactic acid and the way in which an enzyme affects metabolism. Metabolomic analysis is a technique for bringing to light information about the types and quantities of the 1,000 or more low molecular weight compounds believed to be present in the cells of living organisms (the metabolome). Our research team further advanced this technology with the development of dynamic metabolomics, which is a technology capable of observing changes in the quantity of metabolites over time (turnover), and has used it in our research. Based on the results of our analysis, we carried out the designed metabolic modifications using genetic engineering, and brought out the full potential of cyanobacteria as microorganisms for use in bioproduction. We hope that this outcome from our research will become a key technology in efforts to establish a process for manufacturing D-lactic acid to serve as a raw material for biodegradable plastics, thereby helping to build a sustainable, low-carbon society.
The other focus of our research is yeasts. Phenols are important hub compounds used in the synthesis of a wide variety of products, including phenolic resins, synthetic fragrances, agrochemicals, and detergents. However, we depend on fossil resources to make most industrially produced phenols, so the creation of substitutes by means of biomanufacturing is eagerly awaited.
In the course of our work to date, our research team has created a phenol-producing strain of yeast by introducing a phenol biosynthesis pathway gene to a strain of the yeast Pichia pastoris capable of efficiently producing tyrosine (a tyrosine chassis), which is a precursor compound in phenol production. Using the fed-batch culture method of continually adding nutrients to the culture medium, we also succeeded in producing phenols. This represented a world first in the production of biophenols from a yeast host.
If phenols or other hydrophobic compounds build up in the culture medium in high concentrations, they are toxic to the microorganisms, inhibiting microorganism growth and reducing material productivity. Accordingly, using a hollow fiber membrane, we developed a phenol pertraction system to extract the phenol produced by microbe fermentation from the culture medium. By combining this system with a culture system, we succeeded in roughly tripling biophenol productivity. We believe that this research achievement will enable us to aim for the establishment of a continuous biophenol fermentative production system. We see potential for the technology developed through this research to also be applied to the fermentative production of a variety of compounds that are highly toxic to microorganisms, and to the separation of fermented products.
Metabolites of microorganisms that regulate our gut flora
We are also researching gut flora, with a focus on the metabolites of gut bacteria. It is widely known that there are specific microorganisms that improve the gut flora environment, but our research team is exploring the metabolites of microorganisms that help to regulate gut flora. Specifically, we are culturing human feces and will analyze any metabolome that can be identified in the culture medium. We are analyzing a diverse array of stool samples, including those from healthy individuals, in order to search for metabolites that are useful in controlling gut flora, and we are already in the process of finding promising substances.
Cutting-edge biomanufacturing research is not limited to a single microorganism or production of just one substance; rather, it explores the possibilities from all directions. Going forward, we aim to improve target substance productivity by optimizing production pathways with the aid of metabolic modifications, and are keen to move forward with the collection and analysis of experiment data, in order to examine such matters as the culture conditions required for mass production. Although we still have innumerable hurdles to overcome, we intend to steadily surmount them through bold efforts.