Until now, the process from development to supply of vaccines —— our trump card in the fight against infectious disease —— has been said to take at least 15 years. However, vaccines against the novel coronavirus COVID-19 have been commercialized in just one year. Some are a completely new type of vaccine based on mRNA drugs. The realm of therapeutics based on mRNA drugs is diverse, encompassing not only the prevention of infectious diseases, but also cancer vaccines and antibody drugs. The emergence of mRNA vaccines is set to speed up research and development of mRNA drugs.
Special Feature 1 – The Past, Present, and Future of Vaccines How the commercialization of COVID-19 vaccines will speed up development of mRNA drugs
composition by Yumi Ohuchi
The COVID-19 vaccine most commonly used in Japan at present is a type called a messenger RNA (mRNA) vaccine (Figure 1). As of March, two mRNA vaccines have been commercialized: one co-produced by U.S. company Pfizer and German company BioNTech (which is already approved in Japan), and the other produced by Moderna of the U.S. The mRNA vaccines are a new type of vaccine, completely different from conventional vaccines that immunized against infectious diseases by means of attenuated or inactivated (no longer infectious) viruses or bacteria.
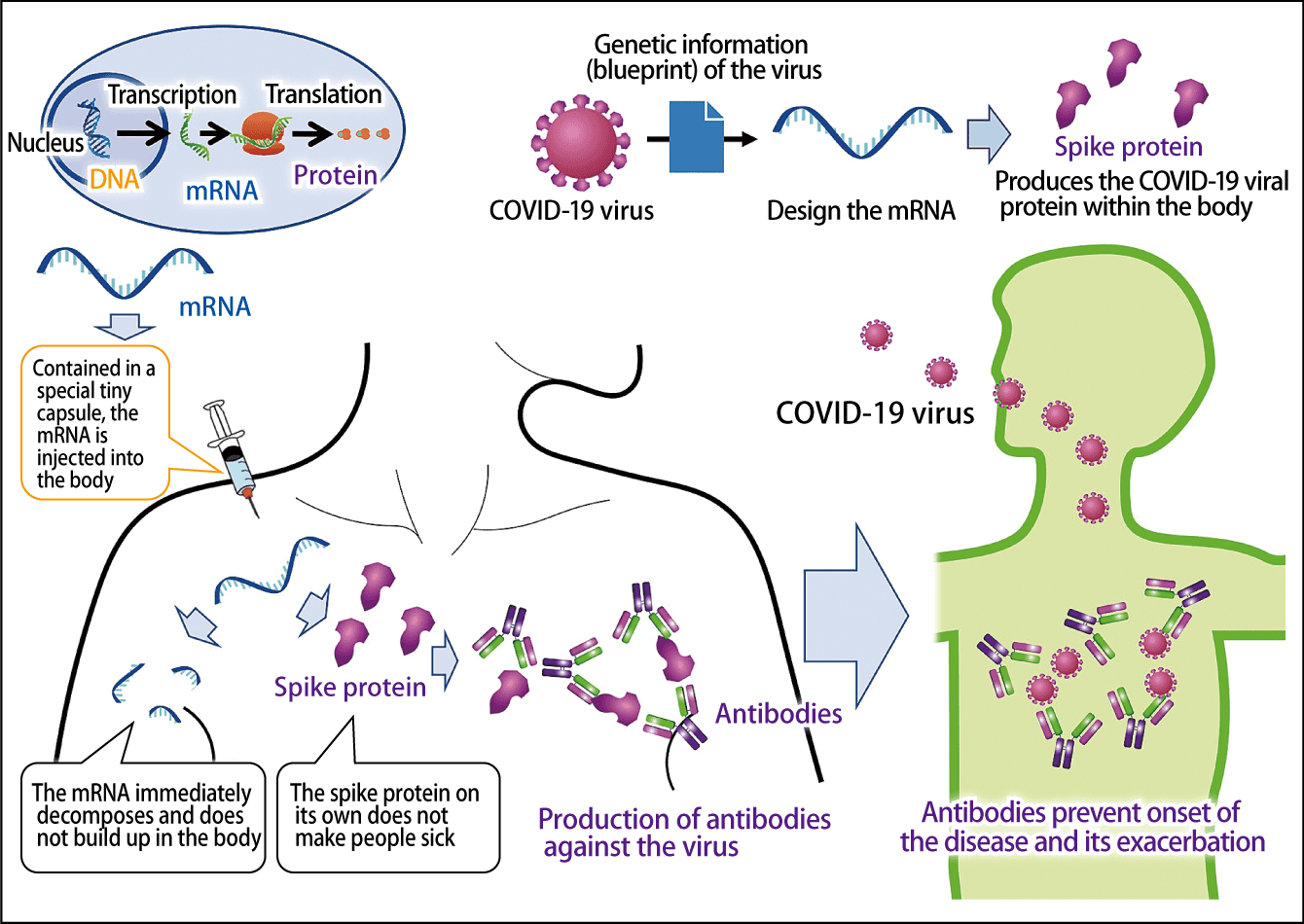
Figure 1. The mechanism behind mRNA vaccinesWhen mRNA designed on the basis of the virus’s genetic information is injected into the body, the immune system kicks in and antibodies to the virus are produced. The virus itself is not administered and neither the mRNA nor the spike protein remains in the body.
mRNA is RNA into which the genetic information carried in the DNA of the nuclei of the body’s cells has been transcribed. As the name suggests, its role is to serve as genetic messenger, carrying information from cell nuclei required to produce proteins. Around 1990, focusing on this function of mRNA, researchers first began to develop mRNA drugs to treat diseases by administering a specific mRNA from outside the body to artificially produce proteins.
The recently developed mRNA vaccines are the first mRNA drugs in the world to have been commercialized. The development of mRNA drugs as vaccines against infectious diseases has been progressing for some time, with clinical trials of vaccines against influenza and Zika virus disease already underway. Vaccine development is usually said to take at least 15 years until the vaccine is available for supply, but mRNA vaccines against COVID-19 have been commercialized in just one year. One reason for this is that these foundations were already in place.
The virus itself is not administered into the body
One key point to remember about mRNA vaccines is that, unlike conventional vaccines, the virus itself is not administered into the body. We know that all coronaviruses, not only the COVID-19 virus, are able to enter the body and cause infection because of spike proteins projecting from the surface of the virus’s cells, which bond to the surface of human cells. Based on the genetic information about the COVID-19 virus, mRNA vaccines insert into the body mRNA designed to produce the virus’s spike protein.
When the spike protein is produced in the body, the immune system recognizes it as a foreign body, causing the production of COVID-19 antibodies, which will neutralize the virus if it subsequently enters the body. This kind of immunity obtained via antibodies is called humoral immunity. In addition, the vaccine also activates cellular immunity, via which immune cells attack cells infected with the virus. These two kinds of immunity exert a preventive effect against infection and acute exacerbation.
The spike protein on its own does not cause infection, and after the mRNA has produced the protein, it quickly breaks down and is eliminated, so it does not affect human genes. In other words, because the virus itself is not used and no damage is caused to human genes, mRNA vaccines are thought to be safer than conventional vaccines.
Whereas conventional vaccines need to be designed for each individual pathogen, mRNA vaccines enable vaccines for other pathogens to be developed using the same design, as long as the genetic information for the pathogen can be analyzed. In fact, as described above, knowledge derived from developing vaccines for influenza and Zika virus disease has been leveraged to address the current pandemic. With regard to COVID-19 virus variants, Moderna has already announced plans to conduct clinical trials of a new vaccine targeting the South African variant. Moreover, we now live in an age in which genetic sequences can easily be decoded. The first cases of COVID-19 were reported at the end of 2019 and Chinese researchers had already sequenced the virus’s entire genome by January 10 the following year.
The side effects and efficacy of mRNA vaccines are also gradually coming to light. Among the side effects of mRNA vaccines are pain at the injection site and other localized reactions, as well as systemic reactions such as fever, fatigue, and headache, all of which are symptoms seen with other vaccines, too. There is a tendency for systemic reactions to be more powerful after the second injection. For example, data from clinical trials of the Pfizer/BioNTech vaccine (10,889 subjects, aged 18-55) showed that 4% experienced fever after the first injection, but this figure rose to 16% after the second. It is thought that production of the spike protein after the first injection causes an immune response after the second, resulting in a fever. In other words, it is regarded as proof that the vaccine is working.
The most serious side effect is anaphylaxis, which can sometimes result in death. Pfizer/BioNTech reported 4.7 cases of anaphylaxis per million doses, while Moderna reported 2.5. Given that anaphylaxis is said to occur in about 1 in 5,000 people administered penicillin antibiotics, it would be fair to say that the risk from these vaccines is lower than in the case of conventional drugs. Moreover, as the onset of symptoms is known to occur within 30 minutes of vaccination in about 90% of cases, it is vital to ensure that people are observed for about 30 minutes after administration and to provide prompt treatment in the event symptoms arise. Another point requiring caution is the fact that people with allergies and a history of anaphylaxis are more prone to post-vaccination anaphylaxis.
In terms of effectiveness, clinical trials of the Pfizer/BioNTech vaccine reported that, when comparing the vaccine group with the placebo group, the efficacy rate (effectiveness in preventing onset of disease symptoms) was 95% from the seventh day after the second vaccination. At 94%, the Moderna vaccine has a similar efficacy rate, demonstrating that both vaccines are highly effective, but we will have to wait and see how long their effectiveness lasts. Data on changes in the number of antibodies after receiving the Moderna vaccine showed that they had only decreased slightly four months after vaccination, so we can expect the effectiveness to last for six months to a year.
mRNA technology enables quick adjustment to variants and scalable supply
As described above, mRNA vaccines are expected to be highly safe and effective, but the shortage of supply is a major issue. As Japan is heavily reliant upon imports from overseas, the supply of vaccines is lower than anticipated, causing delays in the vaccination program. This demonstrates the importance of developing highly safe and effective domestically produced vaccines and of putting in place a system capable of providing a stable supply of vaccines to deal with variants as well.
Accordingly, our research team (from iCONM and Tokyo Metropolitan Institute of Medical Science) has been working on the development of an mRNA vaccine against COVID-19 since April 2020. Most important in the development process is the technology for delivering the mRNA safely and efficiently to cells. As mRNA decomposes immediately after being injected into the body, it needs to be protected until it reaches the cells, something that requires advanced design and technical capabilities to achieve.
In the course of research into other diseases, we had already developed a polymeric micelle called a nanomachine that fulfills this protective role (Figure 2). The nanomachine is a tiny capsule measuring just 50 nm or so, which is so small that it cannot be seen with an ordinary optical microscope, requiring an electron microscope instead. It consists of highly biocompatible polyethylene glycol and polypeptides, which have been confirmed safe in toxicity tests on crab-eating macaques (Macaca fascicularis). After being integrated into cells by means of endocytosis (the process by which substances outside a cell are brought into it), the nanomachines containing the mRNA collapse, releasing the mRNA.
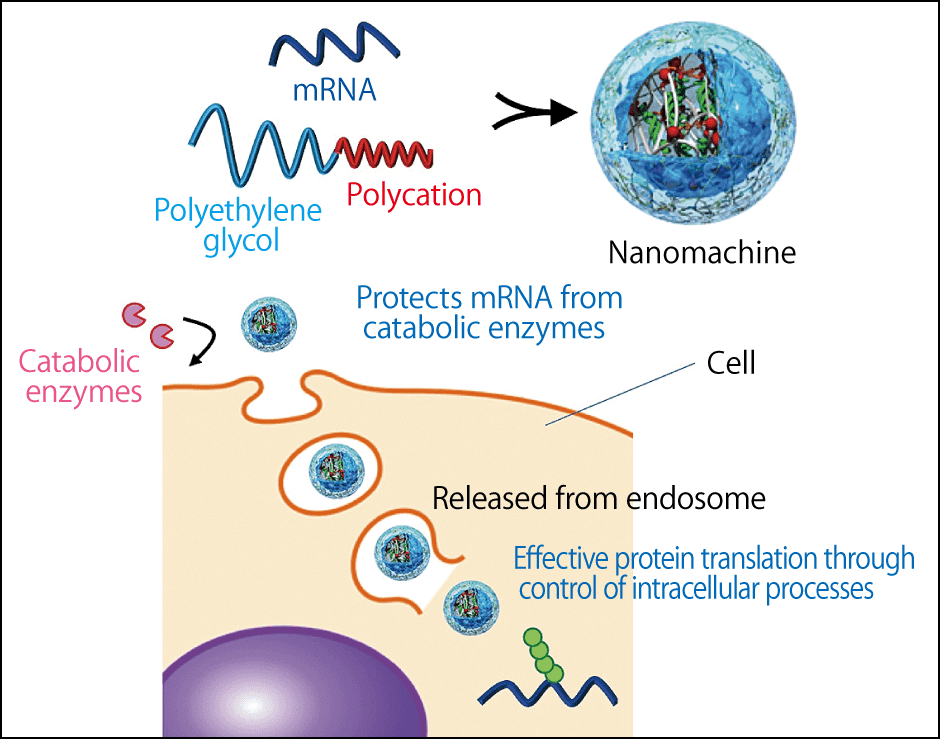
Figure 2. Function of the nanomachineThe nanomachine has been developed with a unique technology different from the first mRNA vaccines, to serve as the capsule protecting the mRNA.
The mRNA released in this way produces the spike protein, but as that alone does not induce immunity, the immune cells need to be activated. In the case of the Pfizer/BioNTech and Moderna vaccines, not only do lipid nanoparticles protect the mRNA, but the lipid component also activates the immune cells. However, as the lipid is a small molecule, it is distributed throughout the body and metabolized by the liver, so there are concerns that immunity could be activated in unnecessary areas, causing an inflammatory reaction. In contrast, our nanomachine is designed to be easily delivered only to the target cells, rather than being distributed throughout the body, based on the hypothesis that we can improve safety in this way.
How are the immune cells activated? We focused on the structure of the novel coronavirus. The COVID-19 virus is a single-stranded RNA consisting of a chain of genetic information, but when it copies itself and proliferates in the cells of the host (a human), it forms a double-stranded structure. The body recognizes these double strands of RNA as a foreign substance and activates the immune cells. In its natural state, mRNA mainly has a single-stranded structure and does not form double strands on its own.
We have already created and repeatedly tested double-stranded mRNA in our past research. One of these studies involved creating mRNA that expressed antigen proteins and varying the structure, so that one type was a single-stranded mRNA, another was a full-length double-stranded mRNA, and another was a partially double-stranded mRNA. Each type was then administered into cultured immune cells from mice. The results showed that the antigen protein production efficiency of the single-stranded mRNA was high, but its immune activation effects were low, whereas the full-length double-stranded mRNA had a high immune activation effect, but a low antigen protein production efficiency, which meant that it did not function as mRNA. We discovered that the partially double-stranded mRNA had the optimal structure, maintaining both mRNA and immune activation functions (Figure 3). When we injected the partially double-stranded mRNA into mouse inguinal lymph nodes, we found that immune cells in the lymph nodes were activated, enabling us to induce both humoral immunity and cellular immunity. We also succeeded in activating immune cells extracted from humans and confirmed that there was no toxicity at the mouse level.
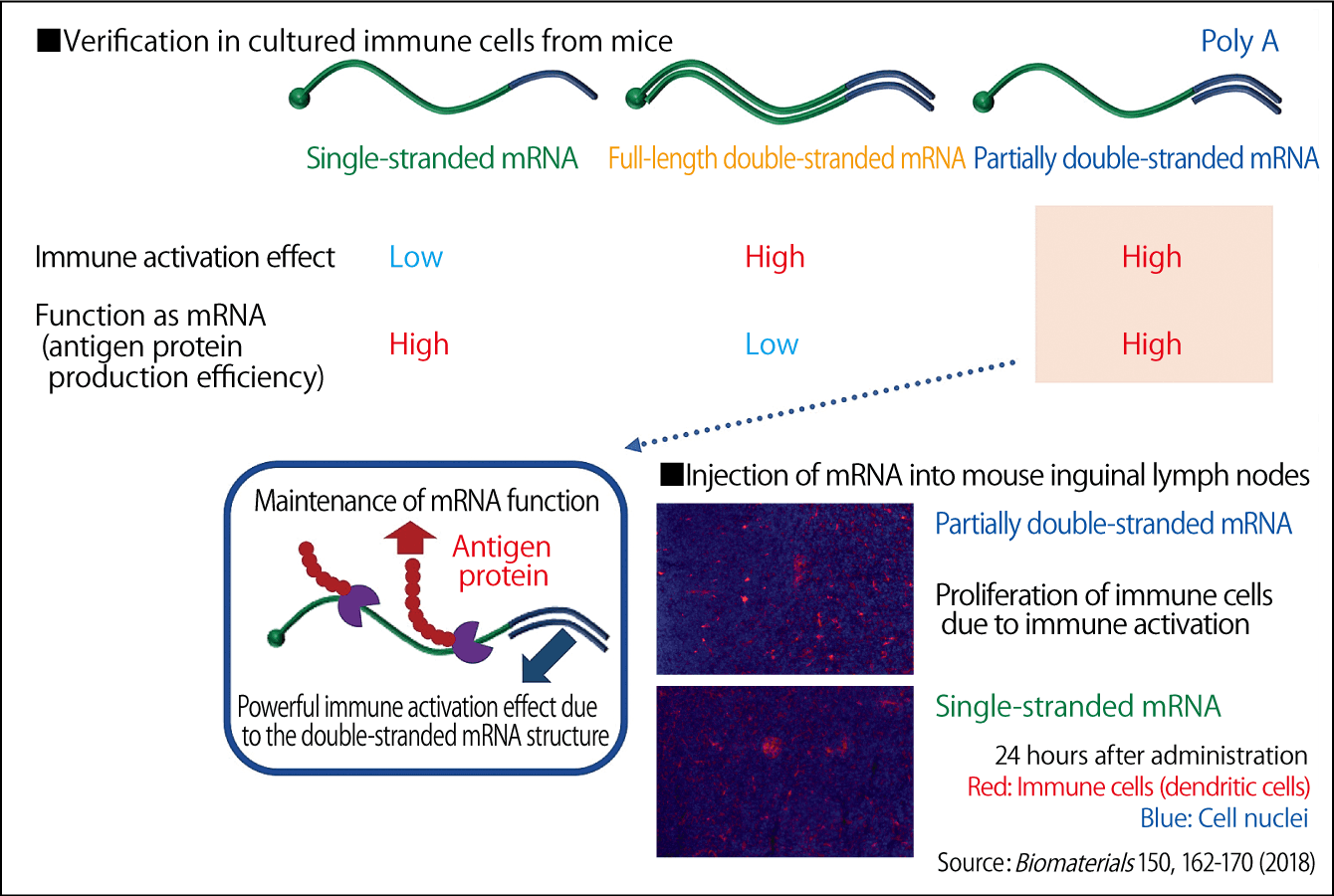
Figure 3. Verification of the most effective mRNA structureThe adoption of a partially double-stranded mRNA structure resulted in both the production of protein serving as an antigen and the immune activation.
We aim to develop a safer, more effective mRNA vaccine that taps into synergies between partially double-stranded mRNA and our unique nanomachine technology developed on the basis of this research. The higher the effectiveness, the less mRNA is needed per dose – making it easier to provide a sufficient supply. In addition, the first mRNA vaccines developed have to be stored at subzero temperatures, but we have succeeded in freeze-drying nanomachines, and if we can apply this to vaccine production, it will enable the vaccines to be stored at room temperature.
R&D of mRNA drugs is stepping up a gear
Our current goal is to complete our animal testing in 2021 and move on to clinical trials. Although vaccination may be progressing, the current situation is that development cannot easily be undertaken as swiftly in Japan as it can in the West. However, by putting in place a sound technology base here, we will be able to apply our technology to variants, new infectious diseases, and infectious diseases for which vaccines have hitherto been difficult to develop, such as respiratory syncytial virus (RSV), which is responsible for many infections in children. As explained at the beginning, therapeutics based on mRNA is not solely focused on infectious diseases. For example, research is progressing into cancer vaccines, cancer immunotherapy, genome editing, antibody drugs, treatments for rare genetic disorders, and protein replacement therapy. The commercialization of the new mRNA vaccines will likely speed up research and development of mRNA drugs.
Cancer vaccines will enable the body to attack cancer cells based on immunity induced via the injection of mRNA that creates proteins expressed by cancer. These vaccines are expected to be able to maintain long-term effectiveness via acquired immunity. Numerous companies are currently undertaking clinical trials targeting a variety of solid cancers, malignant melanoma (skin cancer), and leukemia, among others. We, too, are embarking on cancer vaccine research using our nanomachines and partially double-stranded mRNA.
We have already undertaken basic research into various diseases. In our mouse experiments, we used mRNA to introduce a genome editing tool (CRISPR-Cas9) into the brain and succeeded in efficiently editing the genomes of brain parenchymal cells (cells that handle the major functions) and neurons. We believe the technique can be applied to treating hereditary encephalopathies. We have also proven that injecting mRNA that produces antibodies to amyloid-beta protein —— whose deposition in the brain is thought to be the main cause of Alzheimer dementia —— into mouse brains reduces the quantity of amyloid-beta. Such research findings can also be applied to other diseases by changing the cells targeted. This is a major advantage, and I regard mRNA drug discovery technology as a means of developing systems for creating a variety of therapeutic drugs within the body, rather than simply a way to create drugs.
Unfortunately, Japan is lagging behind the West in the field of research into gene therapies, including mRNA. Among the diverse array of problems behind this are a lack of personnel and funding, but I hope that the development of Japan’s first domestically produced mRNA vaccine will lead to progress in gene therapies and mRNA drugs in the future.