Photosynthesis is the reaction in which plants use light to convert carbon dioxide and water into organic matter and emit oxygen. Thanks to photosynthesis, the organisms on Earth that require oxygen are able to survive and evolve. Accordingly, photosynthesis has been described as one of the best mechanisms to have emerged since life on our planet came into existence. The detailed mechanism had remained shrouded in mystery down through the ages. However, a fragment of that mechanism has now, at last, been revealed. Further studies on the process may lead to the realization of artificial photosynthesis, which will undoubtedly contribute substantially to the combat against global warming.
Special Feature 1 – The Wondrous Survival Strategies of Plants The mechanisms of photosynthesis being brought to light
composition and photographs by Takakazu Kawasaki
Photosynthesis is the reaction in which plants use light energy to produce starches and other nutrients from carbon dioxide (CO2) and water in their chloroplasts and emit oxygen. Most readers will doubtless be familiar with photosynthesis, as it first appears on the curriculum in the upper years of elementary school.
Without oxygen, aerobic organisms such as ourselves cannot breathe and therefore cannot live. Moreover, the starches, sugars, and other organic matter synthesized by means of photosynthesis are essential foods that enable us to live. Even those plants that we cannot eat serve as a source of nutrition for us in the form of feed for livestock and other animals, while fossilized plants sustain our lives as fossil fuels such as oil, coal, and natural gas. Thus, oxygen and nutrients such as starch produced using the sun’s energy via photosynthesis are sources of life for us.
We depend on photosynthesis for our lives
Around 4.6 billion years have passed since our planet Earth formed. Immediately after its formation, the atmosphere covering Earth was enriched in CO2 without oxygen. About 2.7 billion years ago, the planet saw the emergence of organisms called cyanobacteria, which photosynthesized oxygen. These cyanobacteria absorbed light with the aid of chlorophyll and used this energy to split water to produce oxygen. Over the subsequent billion years or so, oxygen has accumulated gradually, and finally reached the level that we enjoy today.
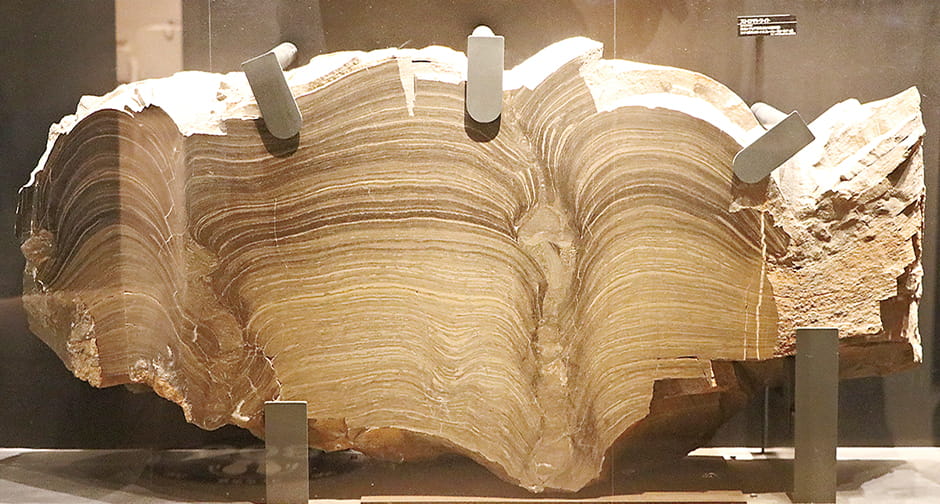
A stromatolite: a layered fossil formed by cyanobacteria, which were the first organisms on Earth to carry out photosynthesis.
Once the level of oxygen (O2) in the atmosphere increased, ozone (O3) was also produced. The ozone layer formed in the stratosphere absorbed the harmful ultraviolet (UV) rays from space to which Earth was exposed, which enabled organisms to move onto land.
Our lives depend on photosynthesis, but it has taken a long time for us to understand the detailed mechanism of how photosynthesis splits water to produce oxygen in chloroplasts.
Our research focuses on how light energy is converted into chemical energy for CO2 fixation via this water-splitting reaction. A protein complex called photosystem II carries out this reaction (Figure 1).
- *1 Photosystem II: An oxygenic photosynthetic reaction system.
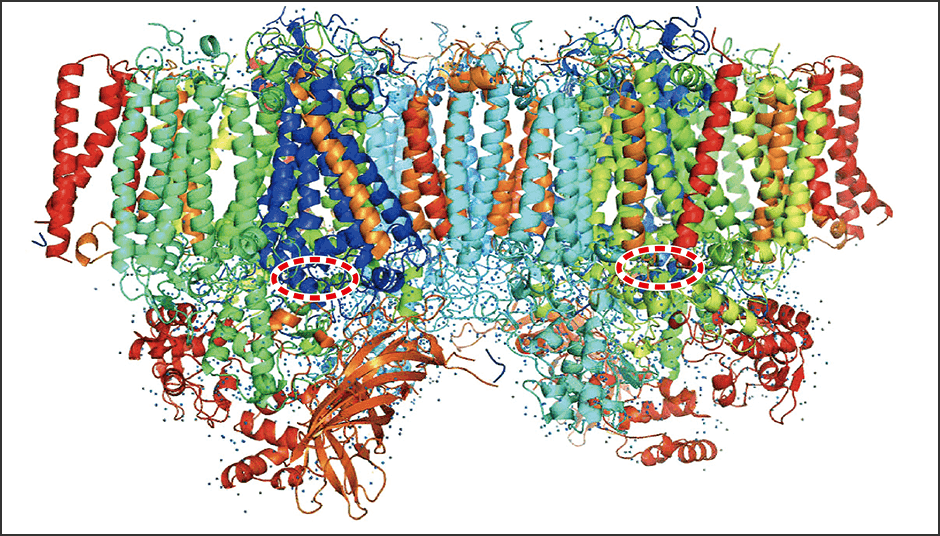
Figure 1. Photosystem II splits waterPhotosystem II, which carries out photosynthesis, forms a dimer consisting of 40 different proteins. Aside from chlorophyll, the colors indicate proteins. The area encircled with the broken red line is a manganese cluster, which is involved in splitting water. Shen and his colleagues published an article on the principles in 2011.
The formula for the chemical reaction that produces oxygen by means of the water–splitting reaction can be expressed as 2H2O -> O2+4H++4e–.
Photosystem II uses light energy to split two water molecules into one oxygen molecule, four hydrogen ions, and four electrons. Then, using the large amount of energy stored in the form of hydrogen ions and electrons (4H+ and 4e–), two high-energy compounds called adenosine triphosphate and nicotinamide adenine dinucleotide phosphate are synthesized. These compounds are in turn used to synthesize organic matter from CO2.
Why do plants’ leaves appear green?
The light used by chlorophyll to carry out this reaction can be identified by investigating the spectrum absorbed by chlorophyll. When visible light rays are dispersed, the colors that appear are, in order from longest wavelength to shortest, red, orange, yellow, green, blue, indigo, and violet. As these are the same colors seen in rainbows, they are also referred to as the seven colors of the rainbow. There are two peaks in the light absorbed by chlorophyll: one around the 440 nm wavelength, in the blue to indigo region, and another around the 680 nm red wavelength (Figure 2). Light is a form of electromagnetic radiation, which has a higher frequency and higher energy at shorter wavelengths. Also used is the term photon, which is a particle consisting of a packet of energy.
- *2 Spectrum: The bands of electromagnetic wavelengths recorded by a spectrograph.
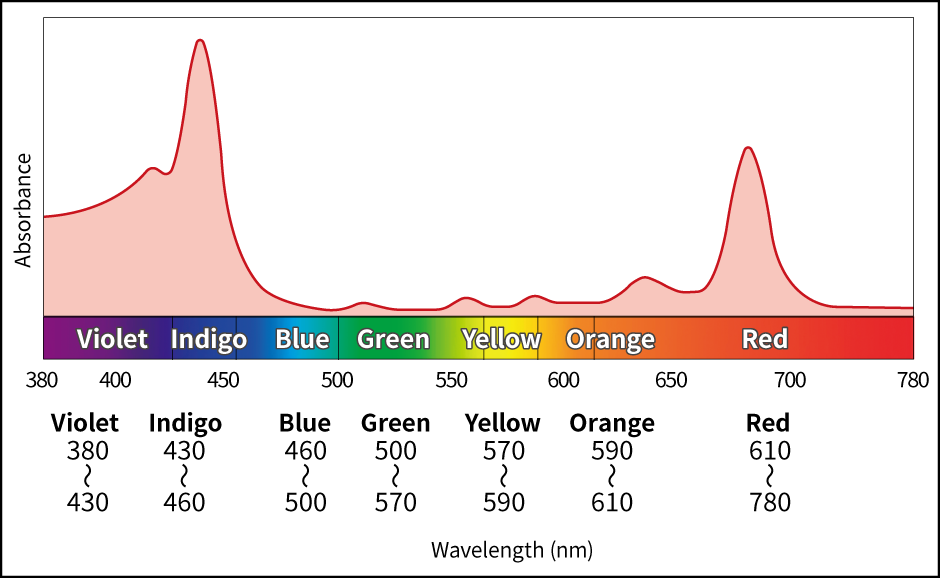
Figure 2. Spectrum absorbed by chlorophyllThere are two peaks in the light absorbed by chlorophyll: one around the 440 nm wavelength, in the blue to indigo region, and another around the 680 nm red wavelength. As chlorophyll reflects light around the green part of the visible light spectrum, rather than absorbing it, the leaves of photosynthetic plants appear green.
In the vegetable factories that have become increasingly common recently, the vegetables are cultivated under LED lighting. Blue and red LEDs are used because they provide light of the most effective wavelength for plant growth.
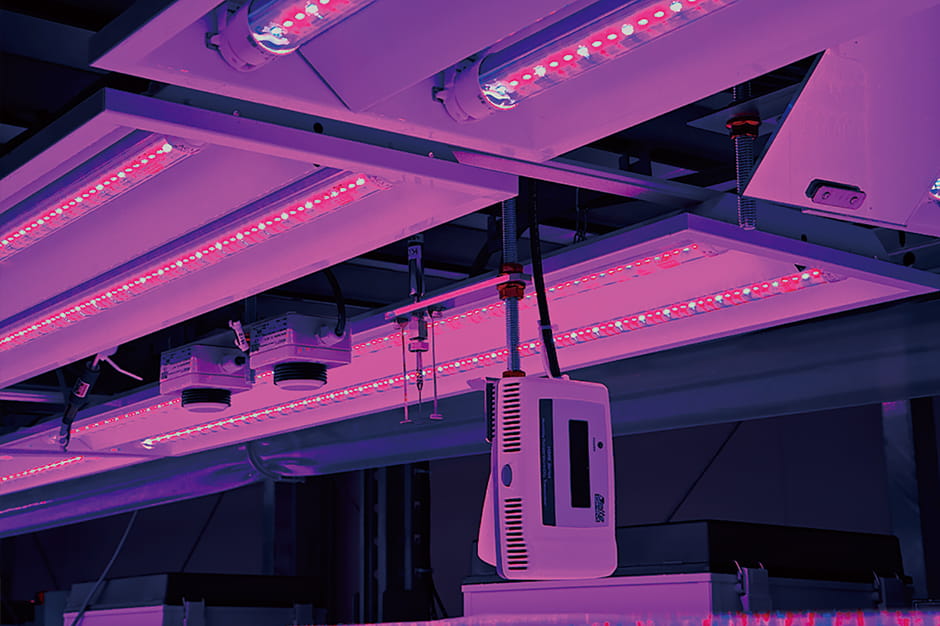
Vegetables being grown under LED light at a vegetable factory. Blue and red LED lamps are used for photosynthesis.
So why do plants’ leaves appear green? Chlorophyll absorbs light with a long wavelength near the red region and light with a short wavelength in the blue to indigo region. Hardly any of the wavelength in the green region —— located in the middle of the seven colors —— is absorbed; these lights are therefore reflected back. That is why plants’ leaves appear green.
Looking at the formula for the water–splitting chemical reaction involved in photosynthesis might have reminded some readers of the electrolysis of water. How is oxygen produced from the electrolysis of water in the absence of a catalyst like photosystem II? Sure enough, just as in photosynthesis, you have to extract four hydrogen ions and four electrons from two water molecules. Four steps are required to extract the electrons in this process, necessitating a great deal of energy —— as much as 4 eV.
- *3 eV: Electron volt. The energy acquired by a single electron accelerating through an electric potential difference of 1 V in a vacuum.
At the long wavelength of 700 nm in the red region, the light energy is 1.77 eV; thus, light alone does not provide sufficient energy, so it cannot split water. However, in the reaction carried out by photosystem II, in the first step in particular, the energy required to extract one hydrogen ion and one electron is low and photosystem II is the enzyme to enable the reaction to proceed adequately even with visible light energy.
The reaction extracting electrons to produce oxygen molecules
A model of the reaction in which the four electrons are extracted has been created. This S–state model begins at S0 and proceeds through four states, from S1 to S4 (Figure 3). In this diagram, “hv” indicates light (photons). In the S0 state, when one photon arrives, one hydrogen ion and one electron are extracted. Next, in the S1 state, when one photon arrives, one electron is extracted, moving it into the S2 state. This reaction continues until, after the fourth time light (a photon) arrives, four electrons have been extracted, releasing an oxygen molecule. In this series of reactions, basically the same number of hydrogen ions and electrons are released, but no hydrogen ions are released in the step from S1 to S2, nor in the step from S3 to S4; instead, two hydrogen ions are released in the final step (S4 -> S0), making a total of four hydrogen ions released.
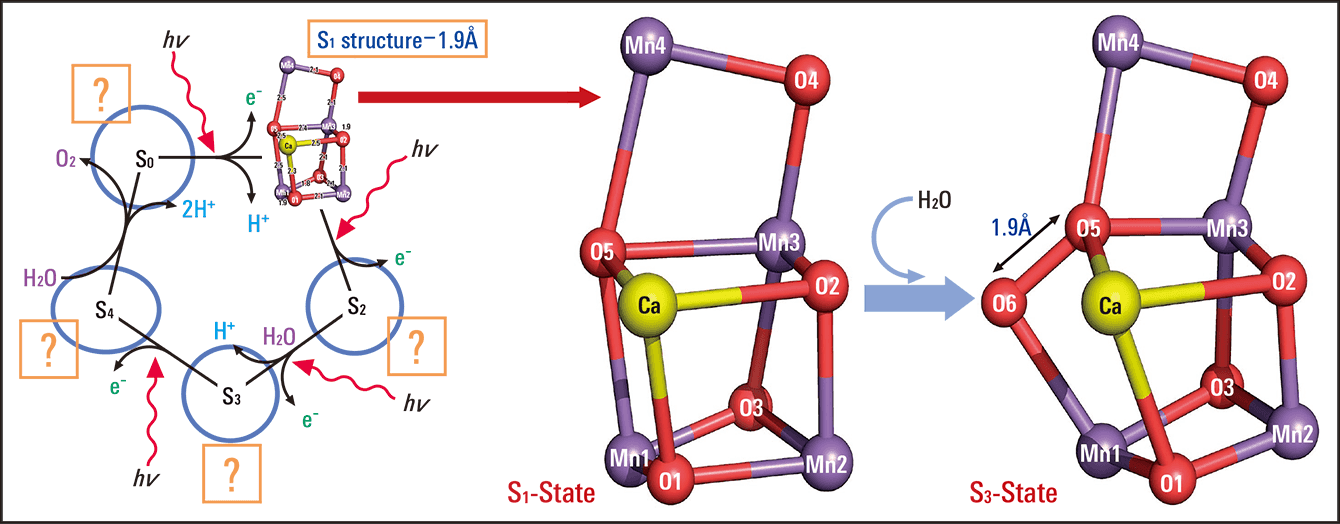
Figure 3. Changes in the S-state in water splittingA model of the S-states (S0–S4) in which four electrons are extracted due to photosynthesis. Once light (a photon) has arrived for the fourth time, four electrons have been extracted, releasing an oxygen molecule. Due to the distorted chair structure, the oxygen atoms closest to each other form a bond to release an oxygen molecule.
This series of reactions was already understood. The catalyst for the reactions was also known to consist basically of manganese and calcium.
What we did not understand was the form taken by the manganese and calcium compound that serves as a catalyst, and the kind of reaction that occurs within this catalyst.
We used a technique called crystal structure analysis to reveal the protein structure of photosystem II. In our research, we found that photosystem II forms a dimer and each dimer was made up of as many as 40 proteins; the different colors in Figure 1 indicate different proteins, while the blue dots are water molecules.
The following reaction occurs in photosystem II. The green chlorophyll molecules absorb light. As light is energy, the chlorophyll emits an electron when it absorbs the light. This leaves the chlorophyll without enough electrons, so it steals an electron from a neighboring molecule. As this reaction continues, the molecule that finally donates the electron to the chlorophyll is a manganese-calcium complex, leaving water as the only molecules able to donate electrons. Thus, the water molecules serve as the electron donor, and after this reaction has taken place four times continuously, two water molecules are split to emit four hydrogen ions and an oxygen molecule.
The manganese-calcium complex in photosystem II is called the Mn4CaO5 cluster, which is indicated in the oval area outlined with a broken red line in Figure 1. We are studying the structure and reactions of this complex.
The manganese-calcium complex consists of four manganese ions and one calcium atom linked by five oxygen atoms. When viewed from the front, it has the shape of a chair, but this chair’s remarkable feature is distortion. Consequently, this is called a “distorted chair” structure. The complex cannot maintain this chair structure on its own, so it is surrounded by amino acids (proteins) to fix it in place.
The complex is distorted because, when the chair structure is distorted and enters a relaxed state, it emits an electron, making it easier for the water-splitting reaction to proceed to the next step. This is a key characteristic and one that we were the first to observe.
To carry out this analysis, it is necessary to expose the complex to light for a very short time, because the reaction will proceed if it is exposed to light for a longer time. To conduct an experiment with a fixed step, we used an ns light pulse. Using an ns light pulse creates a state in which a single electron is released. We then analyzed the structural data of the manganese-calcium complex using the X-ray free-electron laser at RIKEN’s facility called SACLA located in the same campus as the large synchrotron radiation facility SPring-8.
- *4 ns: Nanosecond. 1 billionth of a second.
- *5 X–ray free-electron laser: An ultrashort pulse laser beam in the powerful X–ray region.
When S1 is exposed to light once, we can see the structure of S2 (S2-S1). Similarly, when S1 is exposed to light twice, we can see the structure of S3 (S3-S1). If we take this data and subtract (S2-S1) from (S3-S1), we can ascertain the structure resulting from the change from S2 to S3. Thus, we were able to see from our experimental data that, whereas in S1 O5 (the fifth oxygen atom) and Mn1 (the first manganese atom) in the manganese-calcium complex had been connected, in S3 the chair structure had changed, as O6 (a new, sixth oxygen atom) had inserted between O5 and Mn1 (Figure 3, right-hand side).
One of the best mechanisms in the history of life
We discovered that O5 and O6 are very close together, at just 1.9 Å apart, so when exposed to light once more, these oxygen atoms (O) may form a bond to provide an oxygen molecule (O2). This is thought to be the oxygen released in photosynthesis.
- *6 Å: Angstrom. 10–10 meter = 0.1 nm.
We analyzed the distorted chair structure in an article published in the international scientific journal Nature in 2011, and our achievement was subsequently deemed one of the top 10 scientific breakthroughs of the year by the journal Science.
However, we have not yet observed the reaction in which photosynthesis actually causes oxygen to be released in an experiment. That is why we are continuing our research in this area today.
One of the major goals of photosynthesis research is artificial photosynthesis. While artificial photosynthesis has been successfully carried out in the laboratory with the aid of precious metals and expensive catalysts, the technology has not yet reached the practical level.
Photosystem II, which carries out the water–splitting reaction, has three key features:
1) Highly efficient use of light energy;
2) Appropriate division of the functions of light energy absorption, electron transfer and the water-splitting reaction as well as the efficient coupling among them, and
3) Use of manganese and calcium, which are cheap and abundant on Earth.
Plants developed a mechanism for synthesizing organic matter using water as a source of electrons, thus they have found the best way to sustain themselves since water and sunlight are virtually unlimited resources. This is one of the best mechanisms that have been developed since life first appeared on Earth. If we develop the ability to carry out artificial photosynthesis to produce hydrogen by using cheap, abundantly available manganese and calcium to split water, as in photosystem II, we will be able to use energy without imposing a burden on the environment or emitting CO2 in the way we do when we consume fossil fuels such as oil, coal, and natural gas.
The energy source for photosynthesis is sunlight. The solar energy that shines down on Earth amounts to 120,000 TW per year. Humanity consumes only around 15 TW of energy per year. This equates to just one hour’s worth of the light energy provided to Earth by the sun in a year. In other words, the sun provides us with around 8,800 times as much energy as we need. I am sure you can see from this what a promising energy source sunlight is.
- *7 TW: Terawatt. 1 TW = 1 trillion watts.
If we can shed light on the mechanisms of photosynthesis and become able to perform artificial photosynthesis cheaply and at scale, we could potentially remedy global warming and the other environmental problems that we currently face, and secure an energy source that does not burden the environment.