If we are to fully consider the future of humanity in the context of the 17 Sustainable Development Goals (SDGs), we must first examine the meaning of sustainability and understand its principles. In biology, an ecosystem in which a diverse array of organisms live in harmony with each other is the fundamental principle of a sustainable society. Based on this concept, attention in a variety of fields is increasingly focused on sustainable biotechnology, which involves harnessing the power of organisms, particularly microorganisms.
Special Feature 1 – Microorganisms and Sustainability Leading the way to a more diverse, sustainable world: The evolution of biotechnology
composition by Rie Iizuka
Today, the word “sustainable” tends to be thought of as having the nuance of aiming for the better, but the original meaning of “sustain” is “to maintain the status quo.” When the suffix “-able” is added, it means “able to be maintained or continued.”
Even before the word “sustainable” took on its current connotations, systems in which cells maintain homeostasis and continue living for a long time were known as “sustainable systems” in the field of biology, with the word being used in the sense of “maintaining the status quo.”
Ecosystems in which a variety of organisms live in harmony
Ultimately, the status quo that we want to maintain is a rich human society. The part of humanity that enjoys the benefits of civilization wants to somehow avoid a situation in which changes in the global environment cause it to lose its current affluence. On the other hand, people suffering from famine and conflict are unlikely to want to maintain their current situation. It seems that prosperity for all humanity will not lead to a sustainable world.
The United Nations (U.N.) has set out targets for the future in the form of the SDGs. However, these goals are merely superficial and right now we need to think more deeply about the principles of what a sustainable system actually looks like and how to achieve it. The notion of what constitutes a sustainable ecosystem has long been a topic for discussion in the field of biology, since well before the SDGs were formulated. The closest thing we have to an answer is that an ecosystem in which a diverse array of organisms live in harmony with each other is sustainable. More specifically, an ecosystem consisting solely of simple, solitary organisms cannot withstand external disturbances and will therefore be destroyed. However, an ecosystem will be sustainable if it consists of diverse organisms that can survive by complementing each other, even if the situation deteriorates. Accordingly, I believe that respecting diversity is of paramount importance in building a sustainable society.
Another feature of unsustainable systems is a lack of circulation. For example, global warming caused by CO2 and, more recently, the issue of plastic waste have become major problems for us. Both fundamentally stem from the lack of a cyclical system that considers what will happen to the subsoil resources we have dug up once we have finished using them and wish to dispose of them.
Consequently, there are two key points involved in sustainability. One is respecting diversity. The other is ensuring circulation. I believe that sustainability means building a system in which diverse elements work in harmony with each other and objects circulate.
Electricity-producing microorganisms
Based on this approach to sustainability, we are working right now on sustainable biotechnology. Leveraging knowledge and technologies focused on harnessing the power of organisms to create a sustainable society, we have been looking at all kinds of microorganisms, such as microorganisms that break down environmental pollutants and those that generate methane gas when they break down biomass waste.
One type of organism that we are investigating is electricity- producing bacteria. Everyone is astonished that such interesting microorganisms could exist, but it seems less surprising when you realize that one thing most organisms have in common is that they use the electric potential difference in chemical reactions to acquire energy to live.
First, allow me to explain the respective methods by which humans and electricity- producing bacteria acquire energy.
To acquire energy, humans need to eat food —— that is to say, to ingest organic matter —— and to breathe oxygen. When humans break down organic matter, a chemical reaction takes place in which CO2 is produced, with the electrons generated in this process being passed on to oxygen to create water. Chemically speaking, there is an electric potential difference between the decomposition of organic matter and aerobic respiration, which releases a flow of electrons that captures energy to synthesize adenosine triphosphate (ATP), the compound that provides cells with energy.
However, in some microorganism species, the electrons generated when organic matter is broken down are not passed on to oxygen, but rather flow out through the cell membrane directly to an electrode (Figure 1). In other words, electricity-producing bacteria are those capable of “electrode respiration,” in which electrons generated by the oxidation of organic matter are discharged to electrodes.
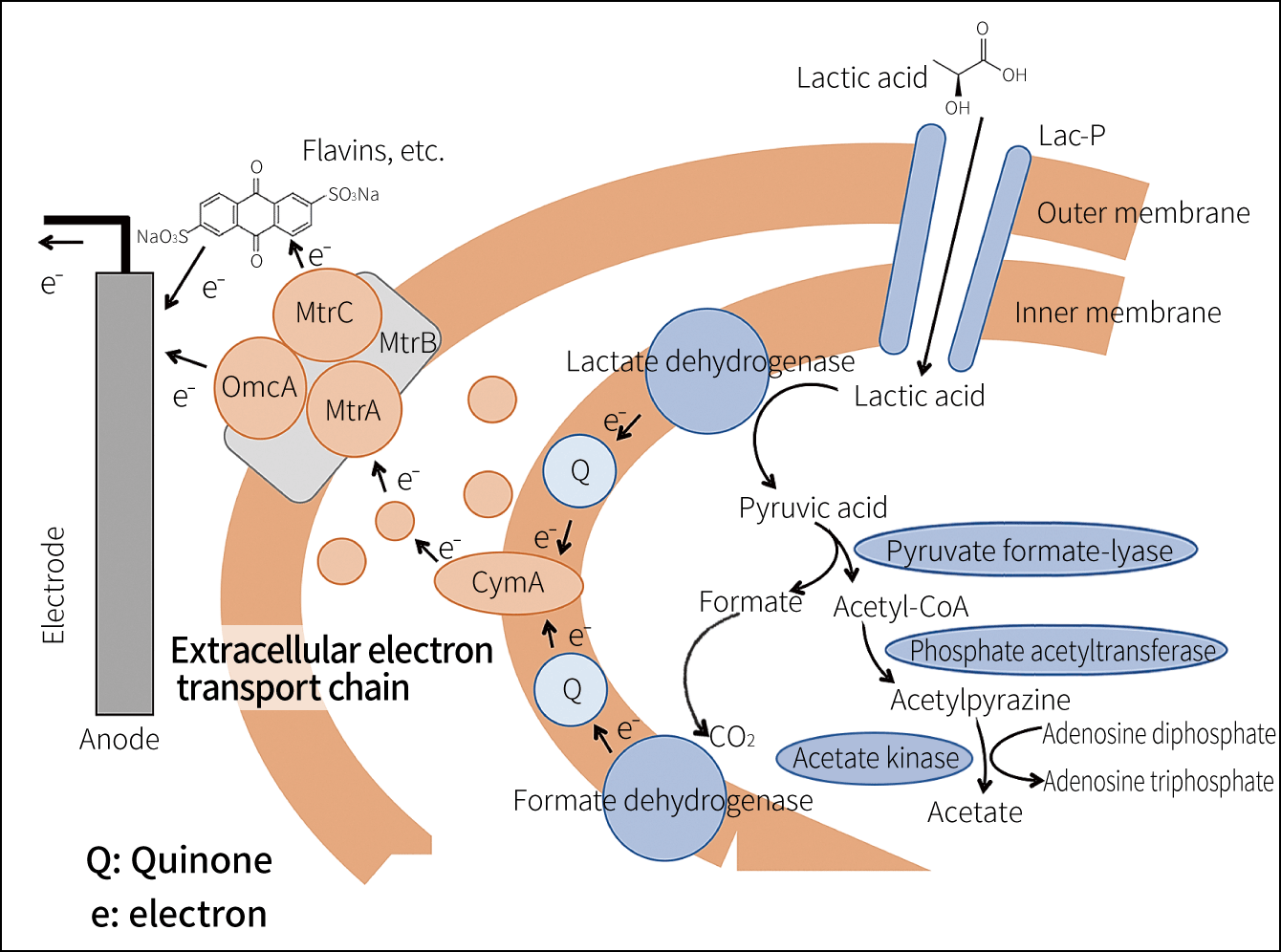
Figure 1. Energy acquisition mechanism of Shewanella oneidensisIn electricity-producing bacteria (electrochemically active bacteria, EAB), electron transport proteins called cytochromes (MtrC, OmcA, MtrA, MtrB, CymA) project outside cells. They play a role akin to electrodes, through which electrons are transferred. Electron transport proteins are an ordinary type of protein also found in humans, but the way in which they are used by EAB differs: they project outside cells and generate electricity. The anode is the electrode allowing current to flow in from an external circuit.
The most famous species of electricity-producing bacteria are Shewanella oneidensis, which was found in Oneida Lake in the U.S., and Geobacter metallireducens, which was discovered in Washington, D.C. (Figure 2). In fact, bacteria that generate electricity to acquire energy can be found surprisingly close at hand in a variety of places, including in Japan’s rice paddies and also in animal intestines.
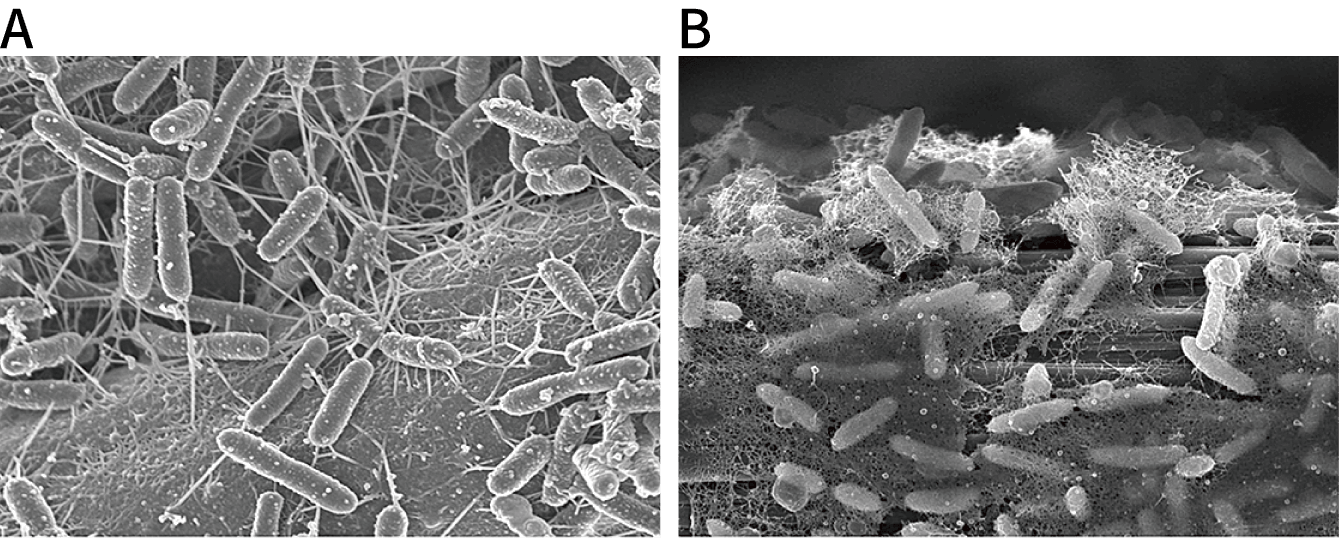
Figure 2. Shewanella (A) and Geobacter (B)The first Shewanella species discovered clings to the surface of minerals at the bottom of Oneida Lake and survives by decomposing organic matter such as fallen leaves and transferring electrons to those minerals. Japanese rice paddies are one of the habitats where Geobacter species have been found.
Electricity-producing bacteria can obtain electrical energy by breaking down organic matter and passing electrons to the external environment, but at the same time, they can receive externally provided electrical energy to promote metabolic reactions., so it is more appropriate to refer to them as “electrochemically active bacteria (EAB)” rather than merely calling them electricity-producing bacteria.
It used to be thought that there were two ways via which organisms obtained energy: chemical synthesis, as in humans, and photosynthesis, as in plants. However, the discovery of EAB brought to the fore the concept of electrosynthesis by means of electrical energy. This is a startling find in the world of biology.
Finding new EAB
Thinking it might be possible to make use of this exchange of electrons between EAB and electrodes in industry and our daily lives, different kinds of devices have been developed.
Microbial fuel cell (MFC)
This device uses microorganisms to produce electrical energy (Figure 3). When the microorganisms are fed with organic matter, they discharge electrons. When these electrons are received by the negative electrode and flow on to the positive electrode, they can rotate a motor or turn on a light. We increased the power generated with the aid of electrodes suitable for the MFC and Shewanella that we had bred, and used them to develop the cassette-electrode microbial fuel cell (CE-MFCs).
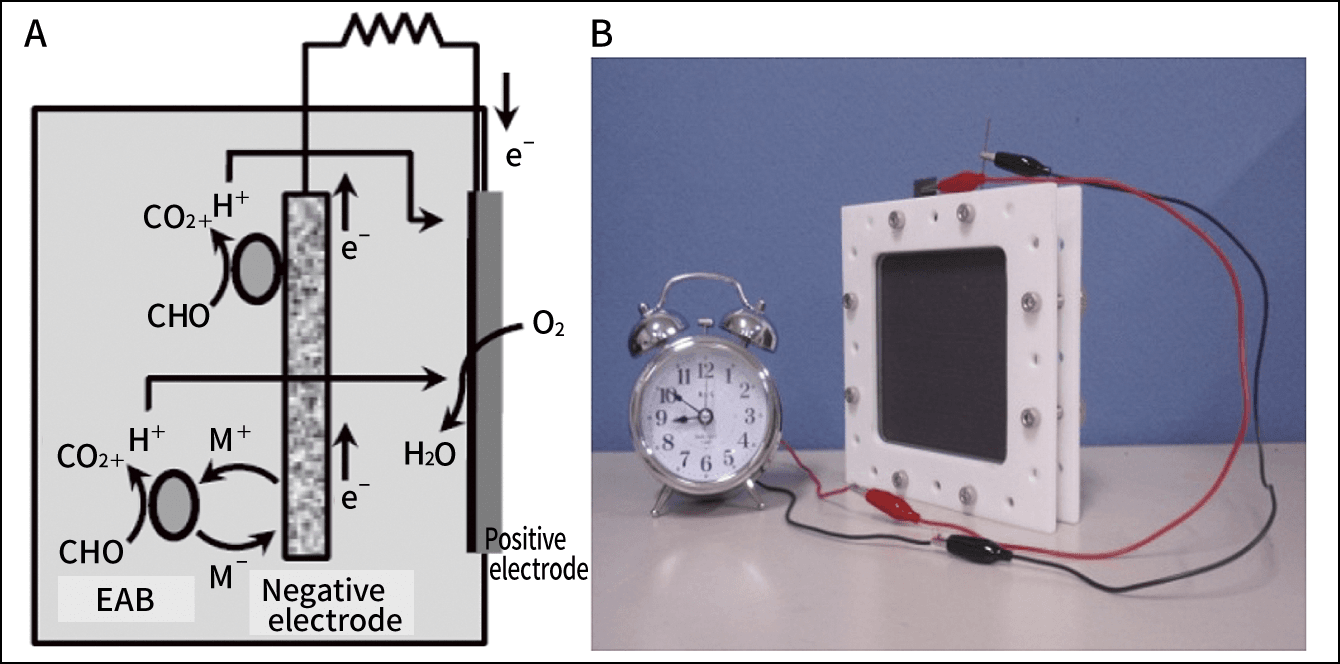
Figure 3. The structure of microbial fuel cell (MFC) [A] and the cassette-electrode MFC [B]This cassette-electrode MFC is about 10 cm square and holds 100 ml. It has an output of 0.3 W, which is enough to power a portable music player.
When wastewater is passed through it, this device can generate electricity while the microorganisms break down pollutants in the water. A major advantage of using microorganisms is that they can generate electricity while treating a diverse array of waste, including household garbage and cattle manure.
Microbial electrolysis cell (MEC)
Applying the mechanism by which microorganisms generate electricity, this device was developed to obtain the hydrogen —— regarded as a clean source of energy —— generated in the process (Figure 4). One of the challenges hindering efforts to encourage the use of hydrogen energy is the cost of its manufacture. There are hopes that this problem could be solved if hydrogen could be produced efficiently from waste. For example, it is conceivable that we could build a cycle in which food waste emitted by large commercial facilities and the like is collected and fed into MECs to produce hydrogen, which would then be sold at a hydrogen refueling station installed at the same facility.
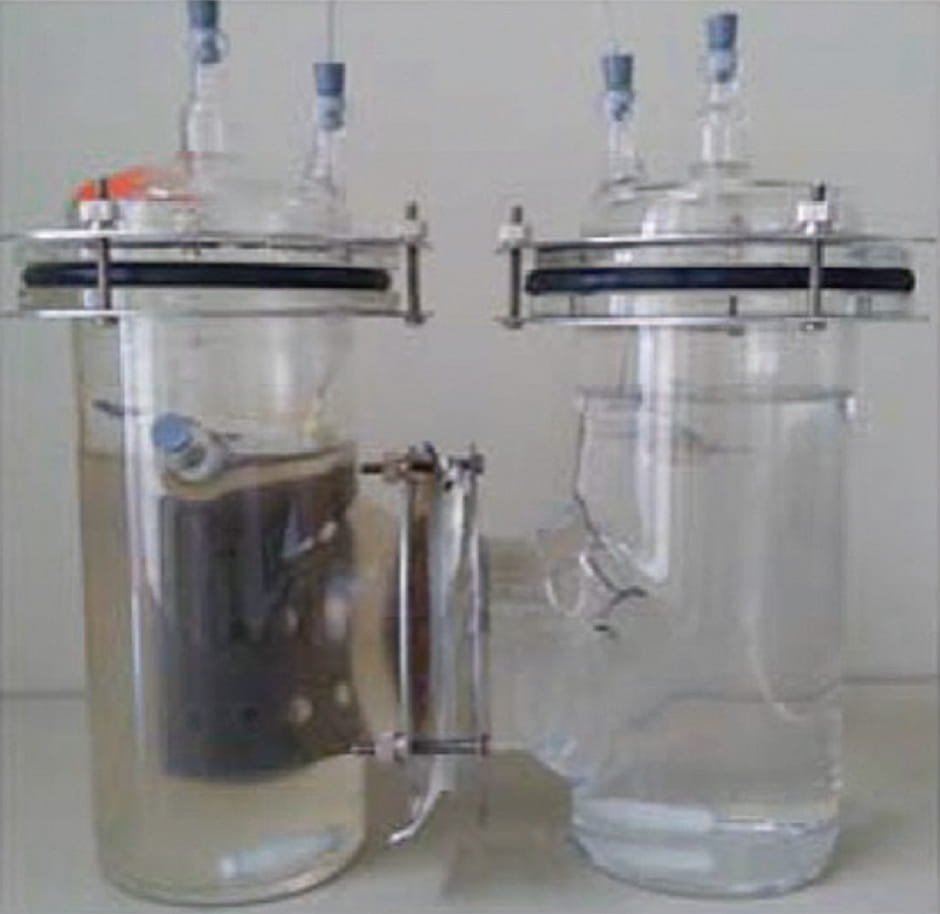
Figure 4. MEC producing hydrogenThe electronic potential generated when the microorganisms break down organic matter is controlled using a potentiostat (a device for controlling electrode potential) and used to reduce protons (positively charged hydrogen ions) in the water to generate hydrogen.
Microbial electrosynthesis (MES)
In contrast to the previous two devices, this device is aimed at synthesizing useful substances by administering electrical energy to microorganisms. For example, one conceivable application would be synthesizing ammonia from nitrogen in air (Figure 5).
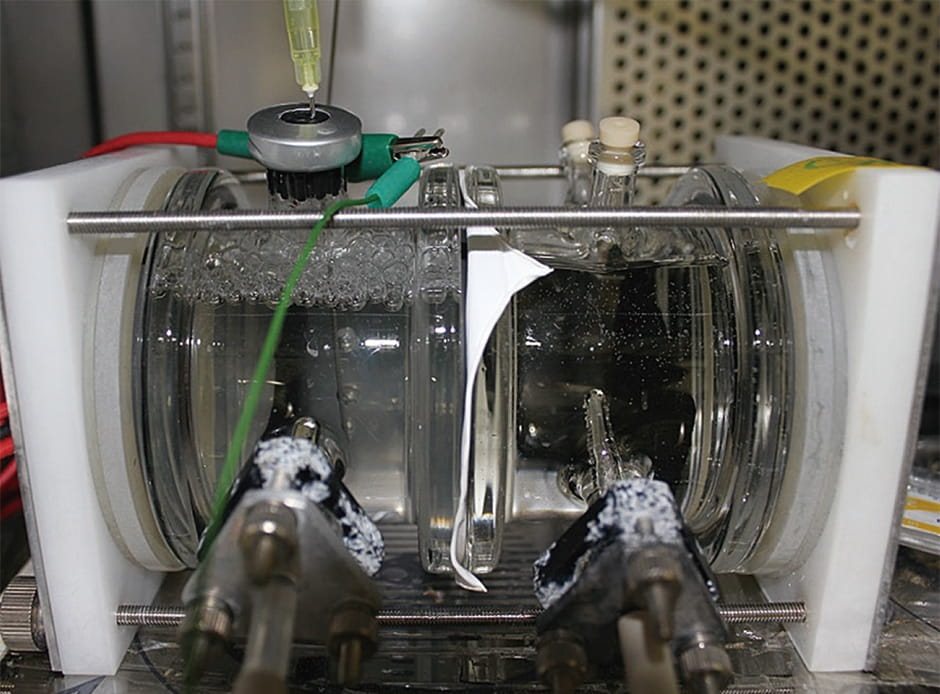
Figure 5. MES synthesizing ammonia from nitrogen in airThis device synthesizes ammonia from nitrogen in air when electricity is administered to Acidithiobacillus. The electrodes provide the necessary electrons. As well as being used in fertilizer, ammonia is attracting attention as a fuel that costs less to manufacture than hydrogen.
As well as considering a roadmap for improving and using devices that utilize EAB, we are currently working with the Miraikan – National Museum of Emerging Science and Innovation on a plan for collaborating with the public to find new EAB. We are going to distribute mud battery kits, which use mud to generate electricity, to people across Japan and have them collect samples of mud at various locations to use in electricity generation experiments. While the first Shewanella and Geobacter species were discovered in the U.S., they were later found to exist in Japan as well. It would be very interesting if we found bacterial species unique to Japan among the soil and mud samples collected by people as part of this project. While conducting experiments with participants to identify the best types of soil and mud, and holding seminars to report on our findings, we hope to build a nationwide network and find new EAB in Japan.
At the same time, another of our tasks is to gain a deeper understanding of the molecular mechanism of EAB. In the Shewanella and Geobacter species mentioned in the examples above, the “electrodes” and “wires” are composed of different types of cytochromes. For instance, whereas Shewanella have 30‐50 types of cytochrome genes, Geobacter have more than 100, each of which is thought to be used for different purposes.
In addition, whereas Shewanella can also live in saline environments and can engage in aerobic respiration in places with oxygen, Geobacter live in rice paddies and other freshwater habitats, and proliferate only in anaerobic conditions. Different species of EAB appear to have different mechanisms for transporting electric potential. For example, there is an electricity- producing type of enteric bacteria that uses a different mechanism from Shewanella and Geobacter. I believe that shedding light on these mechanisms will lead to the cultivation of bacteria with high generating efficiency.
Having diverse options is the quickest route to sustainability
Thus, we are currently exploring ways of using EAB, and I hope that we will be able to contribute to the formation of a sustainable society by achieving their social implementation in various forms. The advantage of using microorganisms lies in their diverse metabolic capabilities. While reaction efficiency of the overall process does not rival that of chemical processes, the fact that innumerable reactions take place simultaneously and complement each other makes it an attractive process. As a result, biomass waste with a complex composition and the like could be used as raw materials for producing electricity or hydrogen, etc. The aforementioned MFCs could be used to generate electricity from waste, and that electricity could then be used to produce useful substances by means of MES. The useful substances produced could then be used by human society before becoming waste again, thereby forming a new cyclical system (Figure 6).
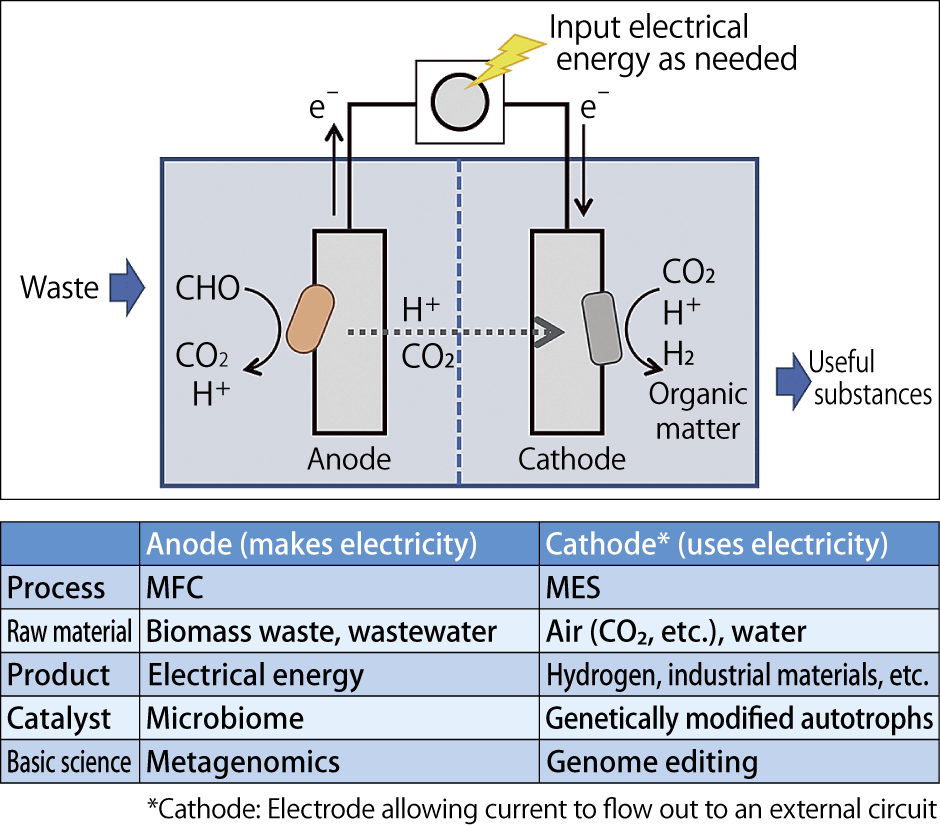
Figure 6. Cyclical system using EAB
I believe sustainable biotechnology should be one of a diverse array of options. While society is now moving toward halting the use of conventional fossil fuels such as oil and coal, such fuels are essentially excellent resources when used in appropriate quantities when truly necessary. While refusing to use petroleum and other fossil resources is extreme and unrealistic, I believe it is important not to over-rely on those resources.
To solve an energy problem, science and technology can play an important role in providing alternative energy sources such as the EAB technique for example. I believe that increasing the diversity of options by incorporating mechanisms capable of functioning at the right time using the right techniques will provide a path that brings us closer to a sustainable society.