It was in the 1960s that hay fever caused by common ragweed (Ambrosia artemisiifolia) and Japanese cedar (Cryptomeria japonica) was first reported in Japan. Since then, the prevalence of pollen allergy has grown year by year to such an extent that it is now called the “national disease” of Japan. The most common form of the disease is Japanese cedar pollinosis, from which approximately 40% of the Japanese population suffers. However, as Japanese cedar——also known by its Japanese name, sugi——is a resource essential to Japan’s daily life and the conservation of its land, the Forestry Agency has been endeavoring to develop low-pollen varieties of the tree. Now, thanks to genome editing technology, improvements aimed at creating a variety that produces no pollen at all are progressing. There are high hopes that humans and sugi trees might be able to coexist.
Special Feature 1 – The Truth about Genome Editing Could producing pollen-free trees reduce hay fever?! High hopes emerge for the coexistence of humans and sugi (Japanese cedar)
composition by Takeaki Kikuchi
Japan is one of the world’s most forested countries, with sugi forests covering approximately 12% of the nation’s land. During World War II and the postwar reconstruction period, extensive logging led to widespread devastation of Japanese forests. To quickly restore the country’s forests, sugi trees were widely planted across Japan. These forests have now reached the point where they should be harvested and replanted. However, due to low timber prices and significant effort and cost associated with reforestation, this process has been slow to progress. As a result of continued release of large quantities of pollen from these mature sugi trees, the problem of Japanese cedar pollinosis (pollen allergy) became apparent. With an estimated 38.8% of Japanese population now affected by Japanese cedar pollinosis, the situation is of serious concern.
Using genome editing to create sugi that produce no pollen
The Forestry Agency has been promoting the spread of low-pollen sugi varieties in several tree breeding projects. Since 2015, we have been working on the development of male-sterile (i.e. pollen-free) sugi lines with the aid of the latest genome editing technology. First, let me introduce some background on what pollen-free sugi trees actually are.
In 1992, a sugi tree that released no pollen whatsoever, despite having male flowers, was found by chance in Toyama Prefecture. This discovery was the catalyst for a survey that found similar sugi in the Kanto and Kansai regions as well; to date, at least 20 pollen-free sugi trees have been identified nationwide. By cross-fertilizing these pollen-free sugi with other sugi varieties, we can produce pollen-free lines of sugi with desirable traits, such as a large tree volume and good stem form, suited for afforestation. However, this will take a very long time.
This is because sugi is an allogamous plant, which means that fertilization occurs between different individuals of the same species. Thus, they are unsuited to self-fertilization, that is cross-fertilization using male and female flowers from a single sugi tree. They need to cross-fertilize with another sugi of a different genetic background. In this respect, the process differs significantly from breeding plants like rice and tomatoes, which are capable of self-propagation.
When a pollen-free sugi tree with normal female flowers is cross-fertilized with a pollen-producing sugi tree, the pollen-free trait does not appear in the first generation of offspring (F1). This is because the pollen-free trait is a recessive trait. Let’s use ‘AA’ to indicate the genotype of a nomal, pollen-producing sugi, and ‘aa’ for the pollen-free sugi. The use of two letters for each genotype indicates that a tree inherits one gene from each parent. All F1 trees resulting from this cross-fertilization will have the genotype ‘Aa’. Since these F1 trees have inherited the pollen-producing gene A, they cannot be pollen-free.
The F1 trees with the ‘Aa’ genotype are then cross-fertilized with each other. The resultant second generation of offspring (F2) has individuals with three different genotypes: ‘AA’, ‘Aa’, and ‘aa’. Only the trees with the genotype ‘aa’ will be pollen-free. The frequency of these genotypes in F2 is approximately 25% ‘AA’, 50% ‘Aa’, and 25% ‘aa’. In other words, pollen-free sugi trees only appear in the F2 generation, with a one-in-four chance (Figure 1). This breeding process typically takes about 10 years. Consideration must also be given to other important traits like growth characteristics suitable for use in afforestation.
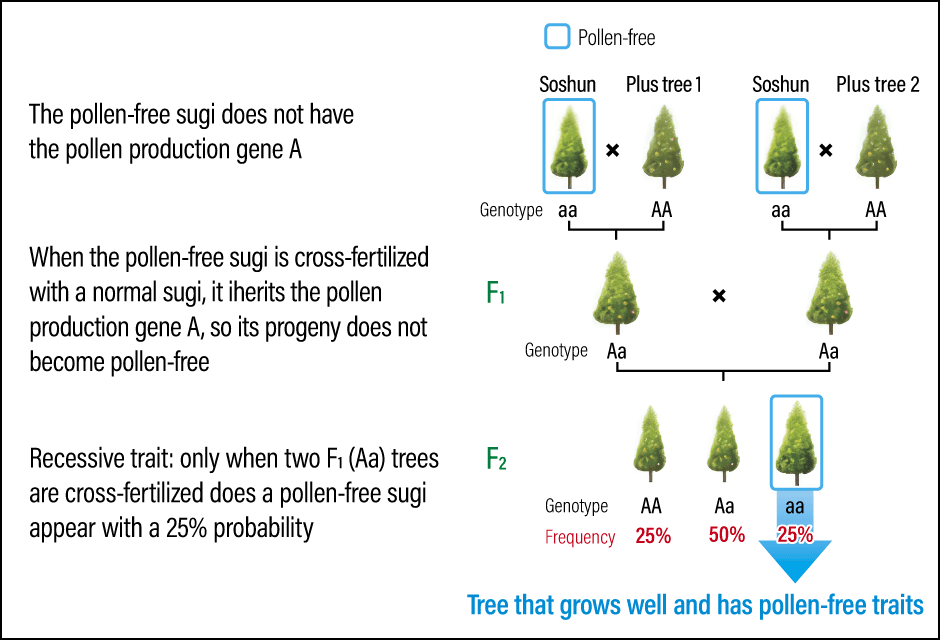
Figure 1. Development of a pollen-free variety using SoshunWhen a pollen-free sugi (aa) is cross-fertilized with a plus tree (AA), the genotype of all the next generation F1 trees is Aa. When F1 trees are cross-fertilized with each other, there is a 25% probability of pollen-free sugi appearing in the F2 generation.
For commercial purposes, we use “plus trees,” —— a tree line with outstanding properties —— for cross-fertilization with pollen-free sugi trees. Plus trees have excellent forestry traits like rapid growth and desirable shape. Efforts to select plus trees began in 1954, and second-generation plus trees —— referred to as “elite trees” —— have been developed by crossing plus trees with each other. By cross-fertilizing plus trees with pollen-free sugi discovered in the natural world, scientists aim to create trees that exhibit outstanding timber characteristics without producing pollen. These selective breeding efforts have been carried out at the Forest Tree Breeding Center of the Forestry and Forest Products Research Institute. The Center has succeeded in developing pollen-free trees by cross-fertilizing a pollen-free sugi named Soshun, which was discovered in the Kanto region, with a plus tree to produce F1 trees. These F1 trees were then cross-fertilized with each other. The resultant pollen-free trees with superior growth characteristics were named “Rin-iku-funen 1.”
The whole genome sequence of sugi has been decoded
We are now working on developing pollen-free sugi using genome editing technology. Genome editing technology is a plant breeding technology that modifies specific gene regions. However, but this technology relies on knowing which region to target. In other words, understanding the molecular mechanisms —— which gene(s) to modify and how —— is essential to produce a pollen-free variety.
The sugi genome is enoumous —— around three times the size of the human genome. Just to give you an idea, the human genome contains around 3 billion base pairs. The sugi genome is also structurally complex, which made it difficult to decode. However, the whole genome sequence of sugi was successfully decoded in 2023 using the latest analysis technology (databases: ForestGEN, BreedingTrees-by-Genes). As a result, scientists have identified approximately 55,000 genes and their locations in the sugi genome, which contains about 9.1 billion base pairs. This breakthrough provides the infrastructure for breeding sugi using its genetic blueprint.
How does genome editing technology produce pollen-free sugi? We focused on the pollen development mechanism. The pollen is contained in the male cones within pollen sacs. Inside these sacs, a layer of cells called the tapetum nourishes the developing pollen grains. In naturally occcuring pollen-free sugi trees, the gene that regulates pollen development in the tapetum has misfunctioned. We aim to mimic nature by inactivating the gene in the tapetum.
Genome editing uses a “scissor protein” composed of two parts: a molecule that identifies the target base sequence and a DNA-cleavage enzyme that cuts the sequence. When the scissor protein enters a cell, it searches for and binds to the gene sequence, then cuts it. However, simply cutting the sequence is not effective. As organisms have mechanisms to repair damaged DNA, the sequence will usually return to its original state. However, in a small percentage of cases, errors occur during the repair processes. Specifically, portions of the sequence may be omitted, rearranged, or altered. This is called a mutation. In genome editing, ensuring that the targeted base sequence remains cut significantly increases the efficiency of generating mutated individuals. Mutations are a phenomenon that can occur randomly in all genes in natural environments. Genome editing allows us to target mutations to a specific gene.
Targeting a gene to cause a mutation
Scissor proteins can easily be introduced into animal cells. In a technique called direct introduction, a scissor protein can be injected into a cell such as a fertilized egg using a very fine needle. After cutting the targeted gene, the scissor protein breaks down and disappears from inside the cell. However, plant cells are much smaller than fertilized animal eggs and are surrounded by rigid cell walls made of cellulose, among other substances. Because this cell wall is very hard, it cannot easily be pierced with a needle. Consequently, direct introduction of scissor proteins is not possible into plant cells.
The method that can be used employs a genetic recombination technique to introduce a scissor gene —— a gene that expresses a scissor protein —— into the plant cell.
Once the scissor gene introduced into the cell is incorporated into the genome, it produces the scissor protein within the cell, causing a mutation in the target gene as a result.
This approach has already been used for breeding of vegetables, among others. To explain it in a way that is easier to understand, I will use as an example the method used to make tomatoes, which are susceptible to disease, more disease-resistant. The scissor gene introduced into the tomato genome by means of genetic recombination and expressed in its cells produces a scissor protein that mutates the disease-susceptible gene into a disease-resistant gene.
Unlike the protein introduced into an animal cell that I mentioned in the explanation of direct introduction above, the scissor gene introduced into the plant cell does not disappear naturally. Therefore, the introduced gene is removed by means of cross-fertilization. When the newly created disease-resistant variety (with the scissor gene) is cross-fertilized with the original variety that was susceptible to disease (without scissor gene), four types of individual are produced: a disease-resistant variety with the scissor gene, a disease-susceptible variety with the scissor gene, a disease-resistant variety without the scissor gene, and a disease-susceptible variety without the scissor gene. From these, the disease-resistant variety without the scissor gene is selected and the series of processes involved in producing genome-edited individuals is completed.
Many countries have prescribed rules on the handling of organisms created with genome editing. Japan requires genome editing technology users to confirm that the organism ultimately has no remnants of another organism’s nucleic acid (DNA or RNA); where no such remnants are present, the organism is not regarded as a living modified organism and may be put on the market once the requisite information has been provided to the competent agencies. The major difference from modified individuals created using conventional genetic recombination is that the introduced foreign gene is not left in the organism. Tomatoes and sea bream are among the foods produced via selective breeding assisted by genome editing that are already sold in Japan. We aim to introduce genome-edited pollen-free sugi into practical use in society at the earliest possible opportunity.
Now let me explain in more detail how the sugi genome is edited (Figure 2).
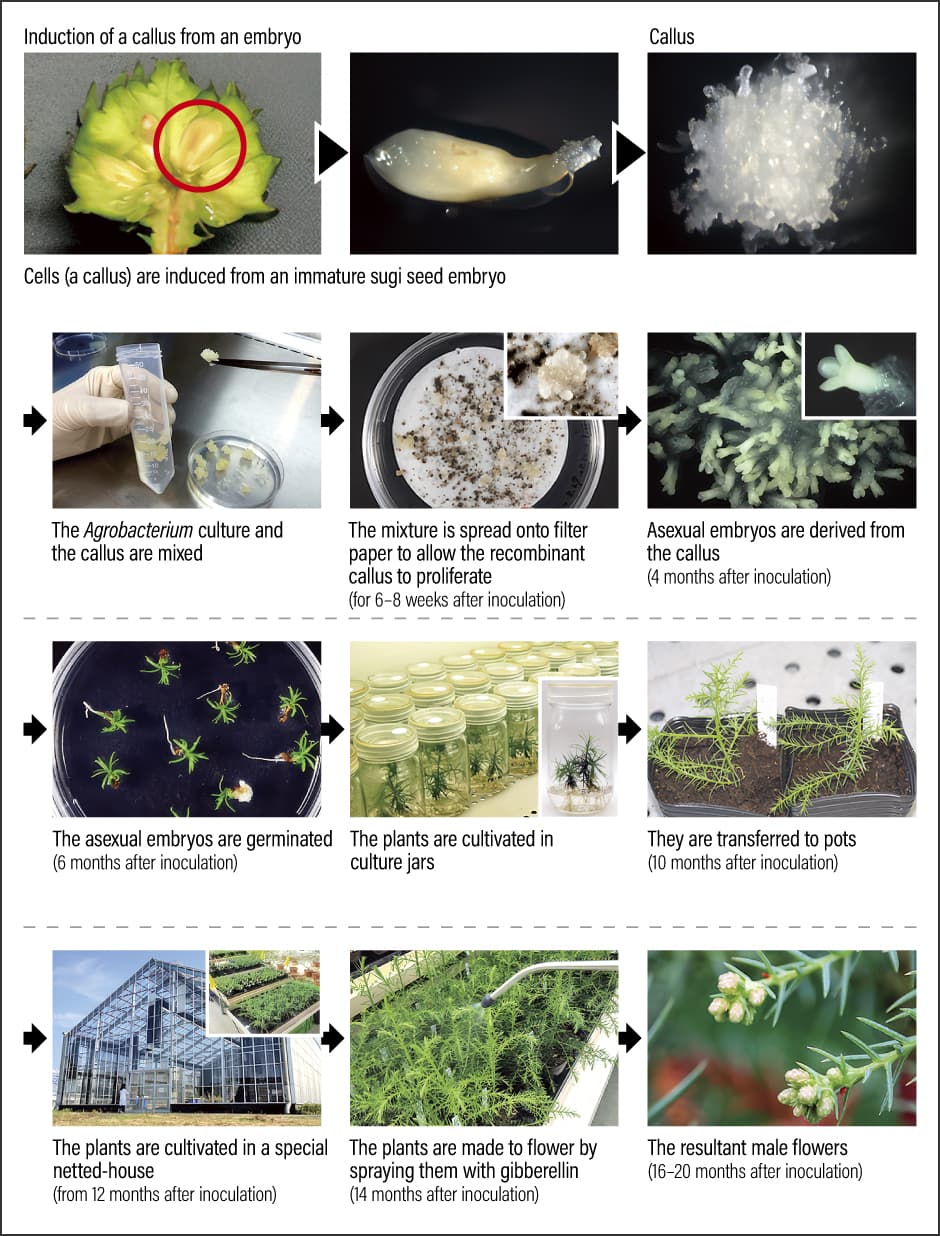
Figure 2. How the sugi genome is editedUsing a bacterial vector called Agrobacterium, the scissor gene is introduced to cells induced and proliferated from a sugi embryo. Asexual embryos are induced, germinated, and cultivated.
First, cells (a callus) are induced from an immature sugi seed embryo using a special culture medium and allowed to proliferate. Then the Agrobacterium that will serve as the gene vector is mixed with the callus, and the scissor gene is introduced into the genomic DNA of the cells. The scissor protein is produced by this expressed gene, causing a mutation in the target gene. Asexual embryos are induced from the callus in which the gene mutation has been completed. An asexual embryo is an embryo produced from a plant’s somatic cells by means of tissue culture technology, without fertilization, and its shape and capabilities are similar to those of a seed embryo. Germinating these asexual embryos produces individuals in which the target region has been modified.
To start with, the germinated sugi are cultivated in the sterile environment of a culture jar. They are then transplanted into growing soil for a while and finally transferred to a special screened greenhouse for ongoing cultivation. A special netted-house is designed to enable plants produced by genetic recombination to be cultivated in an environment close to the outdoors, and is equipped with measures to prevent the pollen, etc. of modified plants from dispersing into the external environment.
Creating improved sugi trees involves more than just eliminating pollen.
Without male flowers, there is no way to check for the presence of pollen inside the male flowers. Sugi trees need to mature before they flower, which takes a considerable amount of time. This is where a plant hormone called gibberellin becomes useful. Spraying trees with gibberellin can induce flowering even in young trees. It can take as little as 16–20 months from the gene introduction experiment to observe male flowers.
Once they are confirmed to be pollen-free, pollen from the male flowers of plus trees is applied to the female flowers of the pollen-free sugi. The F1 trees created in this way are then cross-fertilized to create F2 trees, from which only the pollen-free sugi have had the introduced scissor gene removed —— referred to as null segregants —— are selected and released (Figure 3).
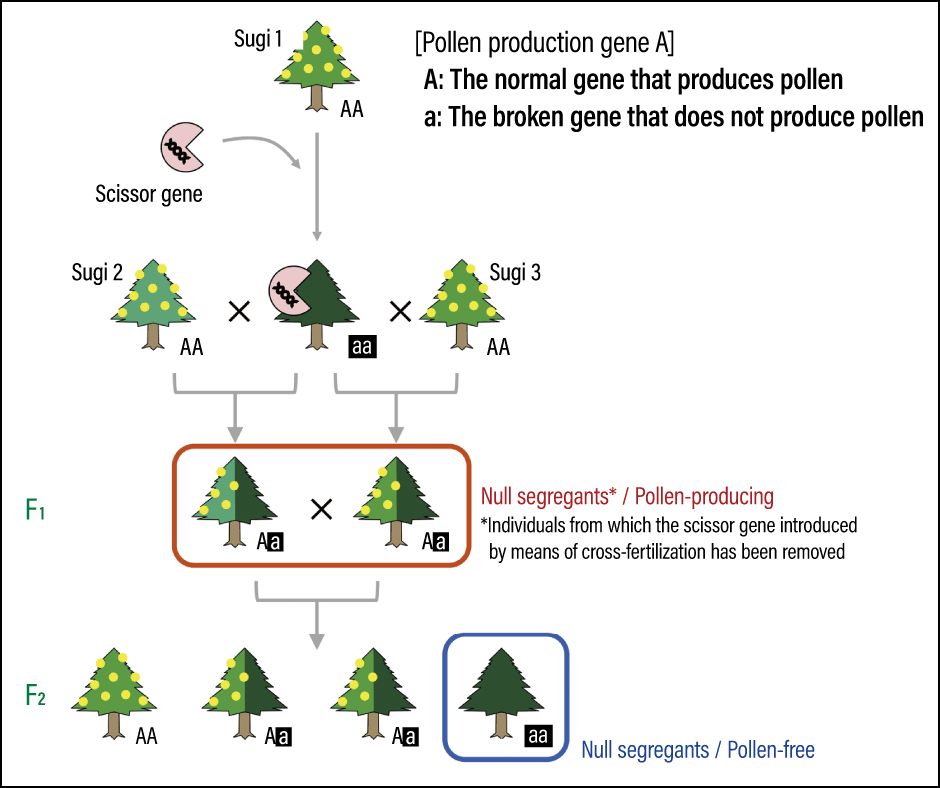
Figure 3. The process of removing a gene introduced by means of cross-fertilizationThe pollen-free sugi to which the scissor gene has been introduced is cross-fertilized with the plus tree. When the F1 trees without the scissor gene are cross-fertilized with each other, they produce F2 trees with neither the scissor gene nor pollen.
To help the public better understand this, we also conduct outreach activities, such as tours of our research facility. In the case of a tour we conducted in November 2021, the proportion of participants who expressed a positive attitude toward genome editing technology increased from 71% before the tour to 95% afterwards. We will continue not only developing plant breeding technology, but also striving to promote public understanding.
Currently, we have high hopes for the development of a technique for direct introduction in plants. This would allow us to introduce the scissor protein itself into plant cells, without genetic recombination. As mentioned earlier, plant cells are surrounded by cell walls. However, research on special cell-penetrating peptides capable of getting through this cell wall is progressing. If we can use these peptides to introduce the scissor protein for genome editing, we will be able to complete the genome editing process without genetic recombination and cross-fertilization, which should reduce the time required. Our research is bringing us closer to establishing a direct introduction-based genome editing technique using cell-penetrating peptides (international patent pending) that we developed in partnership with Tottori University (Figure 4).
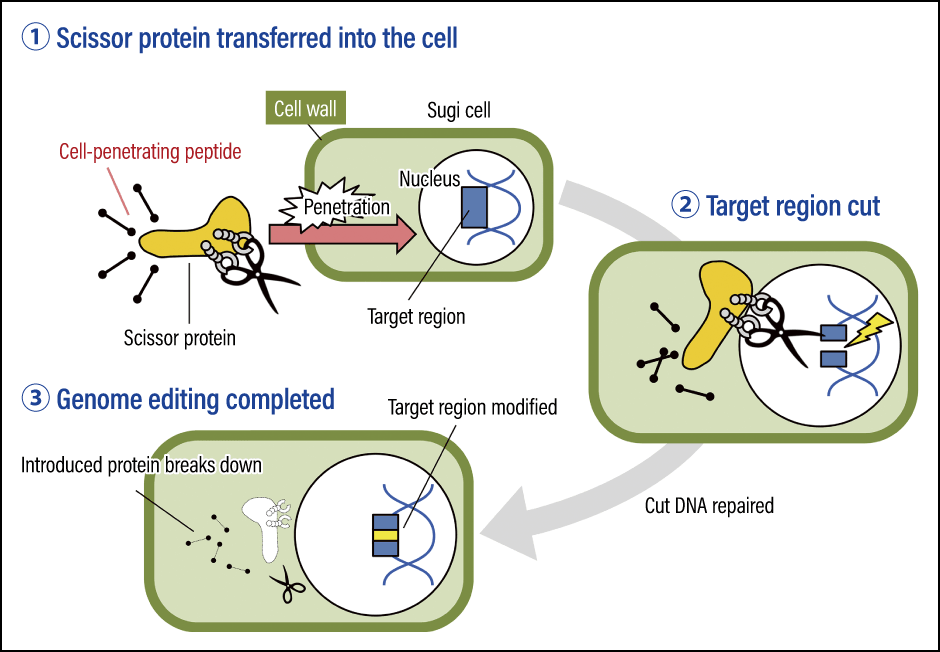
Figure 4. Development of a direct genome editing system that does not involve a genetic recombination stepThe scissor protein is introduced into the cell along with the special peptide that penetrates the plant’s cell wall. After modifying the target region, the scissor protein is broken down naturally.
Improving sugi is more than just creating pollen-free varieties. Traits such as rapid growth, high-quality timber, and increased CO2 absorption are also important. We are eager to use genome editing technology to continue our research in order to produce sugi trees that are not only pollen-free but also highly economically efficient and helpful in preventing global warming.