Newts are renowned for their outstanding regenerative capability, which allows them to repair damaged tissue, but the mechanism behind this ability had long remained unexplained. However, studies using the latest genome analysis techniques have revealed a regeneration gene that is specifically expressed in certain red blood cells. Accounting for around 25% of all red blood cells, these cells circulate throughout the body alongside the other red blood cells. Red blood cells —— also known as erythrocytes —— play a part in gas exchange by transporting oxygen to body tissues and receiving carbon dioxide in return. Scientists are achieving progress in research into newt-type regenerative medicine, which will facilitate human regenerative medicine through the application of this mechanism.
Special Feature 1 – The Mechanisms and Functions of Blood Red blood cells hold the key to hopes for newt-type regenerative medicine
composition by Toshiko Mogi
Living creatures are equipped with regenerative capabilities that enable them to repair damaged tissue. Amphibians in particular are known to have a high level of regenerative ability. For example, even if they have not witnessed it for themselves, most people know that certain types of amphibians can grow back their tail or a limb if it is cut off.
Newts’ capabilities can assist in human regenerative medicine
Amphibian is the collective term for vertebrates that live both in water and on land. While they live in water during their larval phase, most then move onto land before maturing. The best-known examples of amphibians are probably frogs, newts, and salamanders. Of these, the amphibian we use as an experimental model is the Japanese fire-bellied newt (Cynops pyrrhogaster) (Figure 1). Consequently, when I mention newts in this article, I am referring to the Japanese fire-bellied newt.
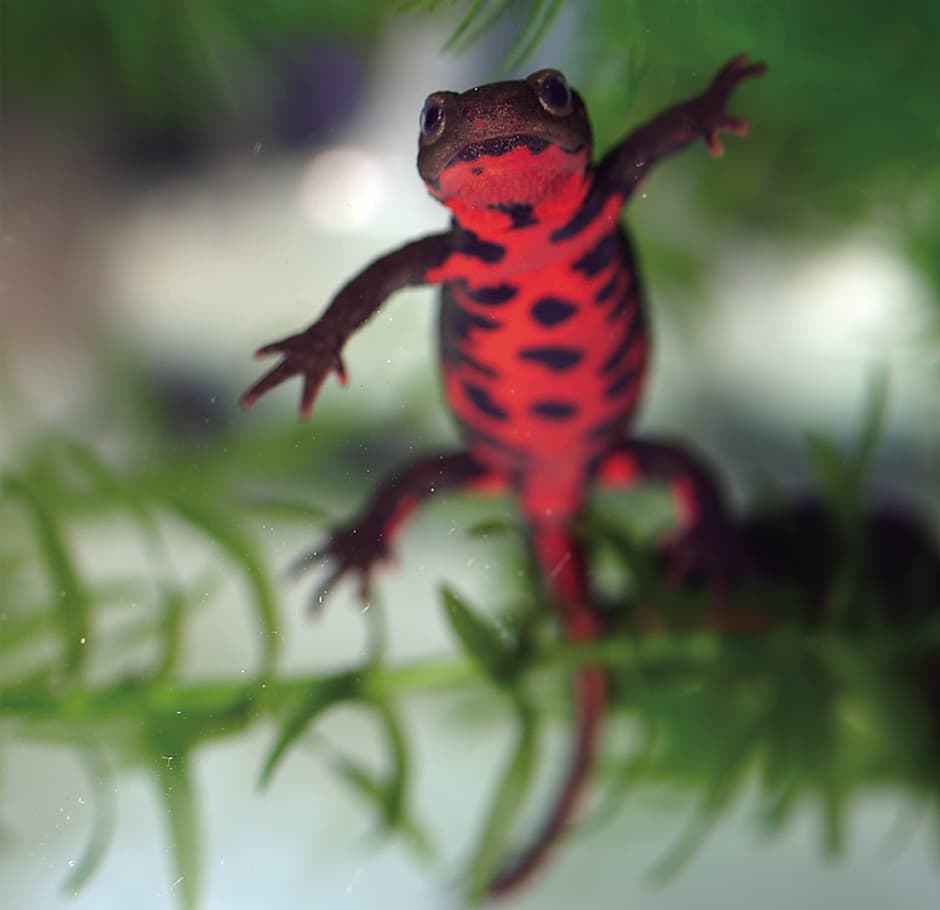
Figure 1. The Japanese fire-bellied newtThe Japanese fire-bellied newt is a species of newt endemic to Japan. It is distributed across a wide range of habitats from flatlands to the mountains, dwelling in rice paddies and ponds with clean water, among others.
But why newts? It is because, even among amphibians, newts are known to have especially outstanding regenerative capabilities. There is an idiomatic expression in Japanese that directly translates as “a lizard casting off its tail,” which is used to describe, for example, a situation in which an underling is made to take the blame for a corporate scandal, thereby enabling senior management to escape responsibility. It refers to the way in which a lizard will spontaneously shed its tail in order to protect itself from an enemy. A new tail will grow back in a matter of months to replace the one that was shed. However, a lizard can only regrow its tail once. Newts, on the other hand, can do so as many times as needed, even once they reach maturity. Moreover, it is not only their tail and limbs that they can regrow: while it might take a little time, if they lose their jaw or even part of their heart or brain, they can regenerate the tissue as new, without leaving any trace of a scar on their skin (Figure 2).
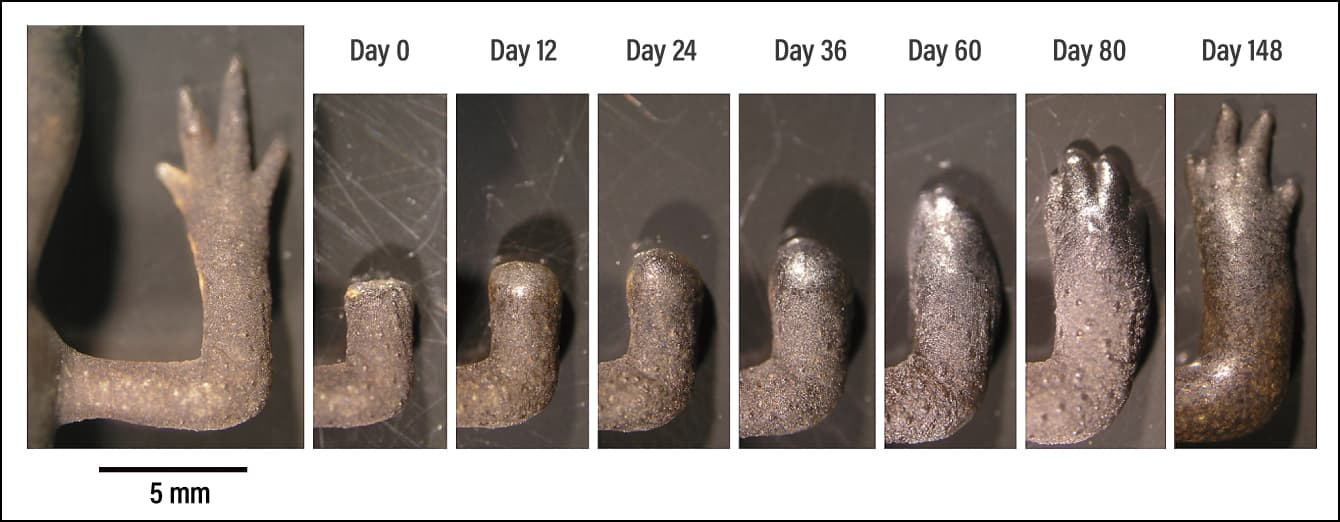
Figure 2. Images of limb regenerationThese images show the process of regeneration after amputation of the normal foot of an adult newt. The stimulus of amputation acts as a trigger activating the genes that bring about regeneration, causing the dedifferentiation of cells near the wound. The first thing to form is a blastema —— a wart-like mass of undifferentiated cells from which regeneration then progresses.
Along with researchers from a number of specialisms, I am engaged in research aimed at putting the excellent regenerative capability of newts to use in human regenerative medicine. In particular, regenerative medical techniques that resolve the issues of fibrosis and scarring are the major challenges we face at present.
The superlative ability of newts to regenerate body parts was common knowledge as far back as 250 years ago, when the science of biology was still in its infancy. However, the mechanism behind this regeneration remained a mystery. While most amphibians have a high regenerative capacity in the larval stage, they lose this ability as they metamorphose and their bodies’ mechanisms and forms change. So, why is it that newts alone can regenerate over and over again, even after metamorphosis? Many researchers thought that newts might actually have pluripotent cells that survived within their bodies.
Focusing on the Japanese fire-bellied newt, whose habitats are found throughout Japan, our research team decided to investigate the regenerative mechanisms of both larval and adult newts. We sought to shed light on whether newts’ exceptional regenerative capabilities are based on a strategy that controls regeneration in larvae or on a novel strategy produced by adult newts after metamorphosis.
The search for the gene that holds the key to outstanding regenerative capability
Using both larval and adult newts as experimental models, we transplanted tissues (skin, bone, muscle, and nerves) that had undergone fluorescent labeling by means of genetic modification onto a limb on both types of model. After the tissue was grafted, we amputated the limbs and investigated which of the tissues in the regenerating limbs the transplanted tissues had differentiated into (differentiation is the phenomenon via which cells that have multiplied through repeated division eventually acquire some kind of specific role). As a result, we discovered the following.
Special cells called stem cells are found throughout the bodies of larval newts. There are a number of different types of stem cells, each of which develops into a predetermined type of cell, such as muscle or bone. If part of the body is injured or lost, these stem cells gather near the site of the wound and transform into muscle or bone cells, for example, thereby regenerating the tissue in its original form.
However, once newts reach adulthood, that regenerative mechanism changes a little. After the limb of an adult newt was amputated, the mature somatic cells at the amputation site lost their properties and functions, temporarily returning to an undifferentiated state. From this, they then proliferated and differentiated anew, turning into muscle, bone, and other cells, thereby regenerating into the original form. The phenomenon in which mature cells return to an undifferentiated state in this way is called dedifferentiation. When you are watching a video and want to rewatch a scene, you can rewind to the point from which you want to watch. Similarly, in the regeneration process in newts, the cells at the site of the injury alone “rewind” to recreate the injured part.
The question is whether newts’ tremendous regeneration ability can be explained solely by the action of genes common to all tetrapods (four-limbed vertebrates), including humans, or whether we have to take genes peculiar to newts into account. This is a fundamental and crucial question in biomedical research aimed at understanding the regenerative capacity of newts and applying it to human medical care.
Our research team has developed its own comprehensive database (named TOTAL) of the genetic (mRNA) information of Japanese fire-bellied newts. Based on the idea that a gene peculiar to newts acts on their regenerative ability, we used this database to search for and analyze the gene that holds the key to this remarkable capability. However, we did not find a regenerative gene specific to newts.
What we did find was a single unnamed gene. We named it Newtic1. Furthermore, when we cross-referenced it with the latest gene databases for a variety of creatures, we discovered that although Newtic1 is not peculiar to newts, it is a unique gene that exists only in Urodela (the order of amphibians that consists of newts and salamanders) and encodes a membrane protein. In order to shed light on which cells express Newtic1, we also produced antibodies to detect Newtic1 protein; as a result of careful scrutiny, we discovered that it is expressed in certain erythrocytes (Figure 3).
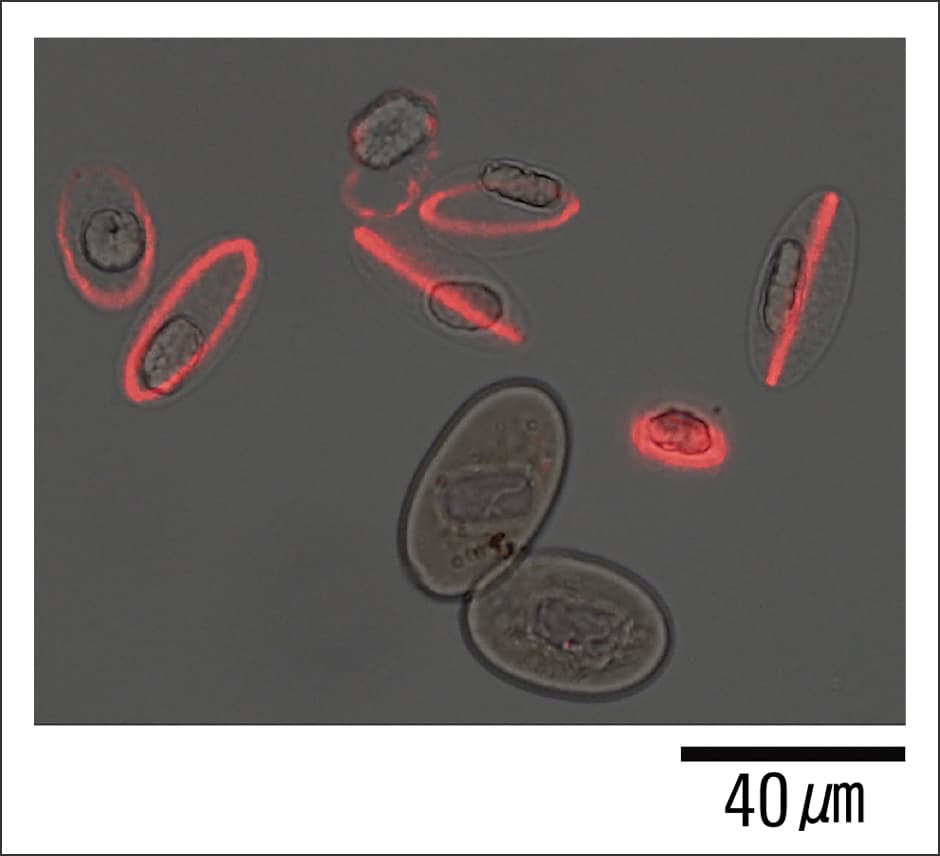
Figure 3. Erythrocytes expressing Newtic1Newtic1 protein is localized in a ring around the edge of erythrocytes that are still in the process of maturing. The two erythrocytes that can be seen below them are already mature and not expressing Newtic1.
Human blood is made in the bone marrow, which is found in the middle of our bones. Hematopoietic stem cells undergo cell division and develop into red and white blood cells, and platelets. In contrast, we know that the blood of adult newts is produced in the spleen and matures as it circulates around the body. When we actually investigated peripheral blood in Japanese fire-bellied newts, we observed erythrocytes at various stages of development.
As a result of even more detailed investigation, we found that Newtic1 is expressed specifically in erythrocytes that are still developing, called polychromatic normoblasts (PcNobs). On average, they account for around 25% of all erythrocytes. In addition, our investigation of normal tissue in adult newts revealed that Newtic1-expressing erythrocytes form small clumps (EryCs), and that in normal blood, these clumps are invariably accompanied by monocytes (a type of white blood cell), forming EryC-monocyte complexes that circulate throughout the body along with erythrocytes that do not express Newtic1 (single erythrocytes that do not form complexes).
Red blood cells are actively involved in regeneration
When we experimentally amputated a limb from adult newts, we discovered that erythrocytes at the amputation site newly expressed Newtic1 in the limb regeneration process and accumulated at the tip of the growing blastema (mass of undifferentiated cells) while forming clumps. In addition, we found that numerous secretory molecules —— including growth factors (such as TGFβ1 and BMP2), matrix metalloproteases, and factors of unknown function —— are expressed within erythrocytes during the regeneration process in adult newts (Figures 4 and 5). In the case of humans, erythrocytes are known to serve as carriers for gas exchange, carrying oxygen throughout the body and receiving carbon dioxide in return. However, in newts, we made an unexpected discovery: erythrocytes are not only involved in gas exchange, but are also actively involved in regeneration.
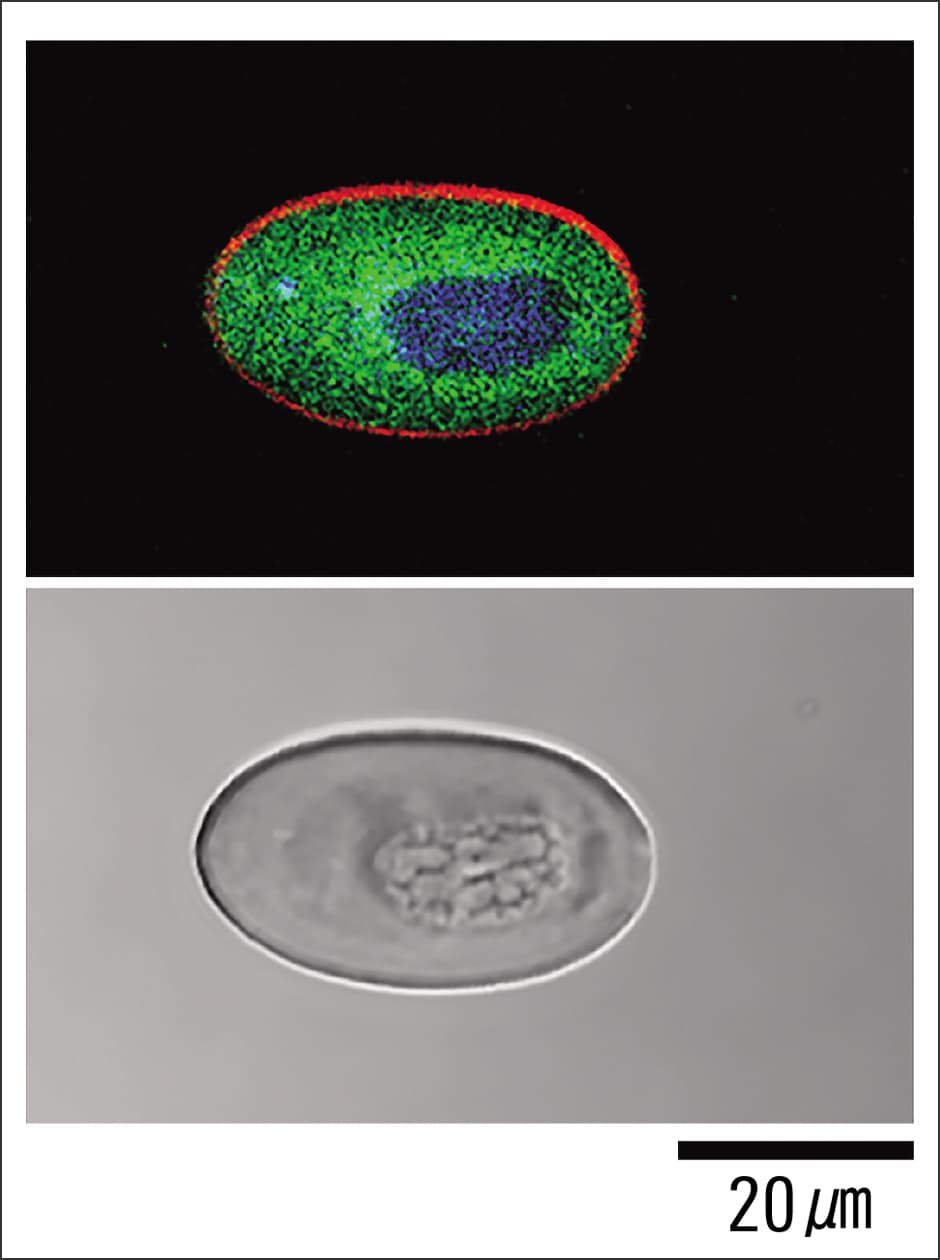
Figure 4. Erythrocyte carrying secretory moleculesDuring the regeneration process in adult newts, erythrocytes internally secrete various molecules associated with regeneration. It is conceivable that erythrocytes are involved in regeneration by acting on other cells and enhancing blood flow via these secretory molecules. In the fluorescence image at the top, red indicates Newtic1, green indicates the growth factor BMP2, and blue indicates the nucleus. The image below it is a bright field image.
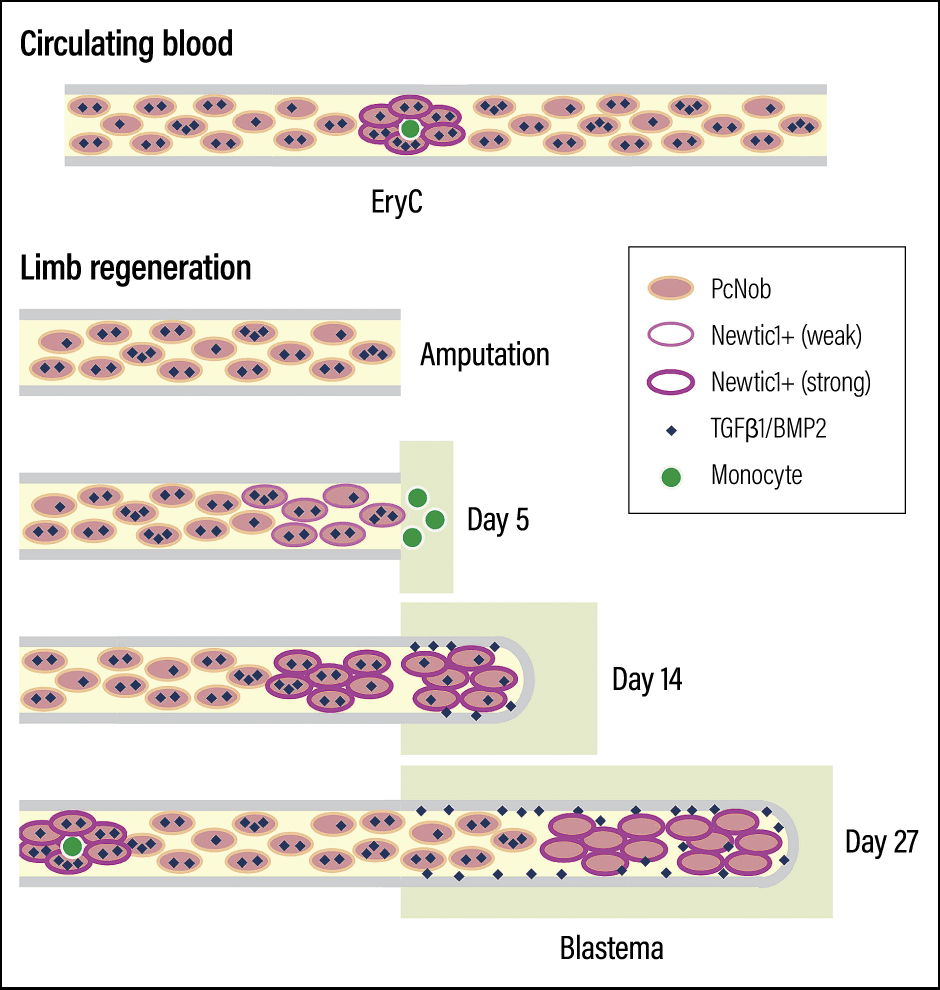
Figure 5. Schematic showing erythrocytes carrying secretory moleculesNewtic1-expressing erythrocytes (PcNobs) form clumps (EryC) accompanied by monocytes (a type of white blood cell: shown in green) in circulating blood. However, during the regeneration process, EryC that are not accompanied by monocytes form and accumulate at the tip. Once blood vessels connect and blood circulation resumes, they are replaced by normal blood.
In a subsequent study, we found that at least 10 key proteins other than Newtic1 are involved in regeneration. When we investigated Newtic1 protein in greater detail, we also learned that it takes the form of small granules. When erythrocytes reach the wound, the proteins required for regeneration enter the Newtic1 granules. Serving as carriers, these granules discharge the proteins associated with regeneration outside the erythrocytes at the site where they are required. We believe this acts like a switch for dedifferentiation, allowing regeneration to take place cleanly, without fibrosis or scarring.
In fact, we ascertained that humans, too, have a substance associated with regeneration that Newtic1 selects and exports from erythrocytes. If humans also have the same substance, why are we not able to regenerate like newts and how would we go about developing that ability? These are the issues we need to address going forward.
When the human body is injured, the first thing that occurs at the site of the injury is inflammation. Fibroblasts gather in the area from surrounding tissue. These fibroblasts then divide, and secrete collagen and other fibrous proteins to harden the wound. This is called fibrosis. The hardened tissue is replaced by tissue with different properties from the original tissue, and remains in the form of a scar.
The regenerative ability of newts “does not become cancerous”
Sometimes, of course, an organ, limb, or other part of the body has to be removed to treat a serious injury or disease. Surgery, such as that performed for cancers of the head and neck, may leave a surgical scar in a visible location. One cannot deny that a visible scar or the loss of a body part can impair the patient’s quality of life. If newt-type regenerative medicine permitted the repair of wounds cleanly, without fibrosis or scarring, one would have to describe it as the ultimate cure.
As is widely known, regenerative medicine encompasses a variety of techniques. In regenerative medicine using iPS cells or ES cells, the stem cells engage in an intensive process of repeated cell division, so the question of how to prevent the cells produced in that process from becoming cancerous poses a major challenge. One thing I would particularly like to note is that newts’ regenerative capability does not result in cancers. In our experiments, we used various carcinogenic substances in an attempt to cause cancer in newts, but the newts did not develop cancer.
Additionally, regenerative medicine using stem cells such as iPS cells requires surgery to transplant tissue or organs produced from stem cells outside the body. However, newt-type regenerative medicine involves regenerating the injured area in its original form, so transplant surgery is not required. Our hope is that this will permit less invasive treatment.
Currently, we are attempting to unravel the factors that will facilitate the newt-type reprogramming of human cells (returning them to an undifferentiated state), in order to perfect a realistic timetable for regenerating damaged human organs in a manner similar to newts. At the same time, we are devoting our energies to making the use of newts more prevalent in a diverse range of medical fields. In our latest study, we have clarified that both the autonomous properties of cells and the extracellular environment that changes due to physical metamorphosis and growth are crucial to the reprogramming of somatic cells in newts.
Moreover, we have demonstrated the importance of newts’ unique blood components and established an experimental system that will enable us to make direct comparisons between regeneration in newts and healing with scar formation (fibrosis) in mice (Figure 6). This study using genetically modified mice and genetically modified Japanese fire-bellied newts represented a major advance, as we replicated the dedifferentiation and redifferentiation process in newts, and established a cell culture system compatible with mammals. Hopes are growing for human regenerative medicine. I am continuing my research, in an effort to make newt-type regenerative medicine a reality at the earliest possible opportunity.
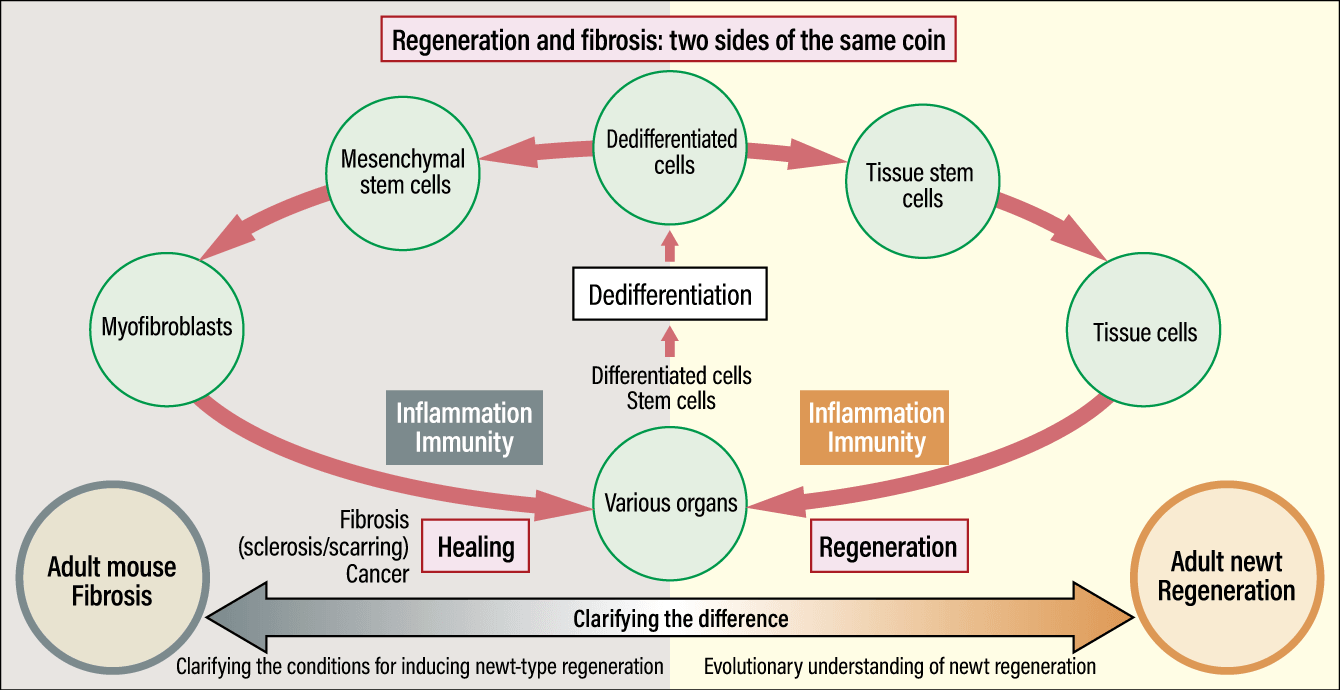
Figure 6. Difference between regeneration in newts and fibrosis in miceDedifferentiation in newts likely evolved from the fibrosis mechanism common to all tetrapods, including humans. By clarifying the difference between the regeneration process in adult newts and the fibrosis process in adult mice, the research team aims to shed light on the evolution of regeneration capabilities in newts and the conditions that will make newt-type regeneration possible in adult mice.