Birds and many other creatures are thought to have an internal compass that detects the Earth’s magnetic field. There are several hypotheses about the nature of this compass in birds. One of the recent —— the radical-pair mechanism hypothesis —— has emerged from the field of quantum biology, which investigates physical phenomena at the molecular level within the body. Efforts to shed light on this mechanism are underway. Photoreceptors in the retina of birds have magnetic properties when activated by blue light and respond to the geomagnetic field. Scientists say these signals affect the birds’ vision, enabling them to perceive direction visually.
Special Feature 1 – Mysteries of Migratory Birds Could a photochemical reaction in the retina make it possible to see the Earth’s magnetic field?!
composition by Yumi Ohuchi
illustration by Rokuhisa Chino
Migratory birds, homing pigeons, salmon and sea turtles that return to the river or sea where they were born-many different wildlife species are able to reach their destinations with unerring accuracy. It is thought that these creatures, in addition to having highly developed senses such as vision and smell, may also have some kind of system that uses an internal compass to obtain information about their location by detecting the Earth’s magnetic field (geomagnetic field) (Figure 1). Given that some migratory birds fly distances of 10,000 km or so without going astray, the association with a geomagnetic compass is considered particularly important.
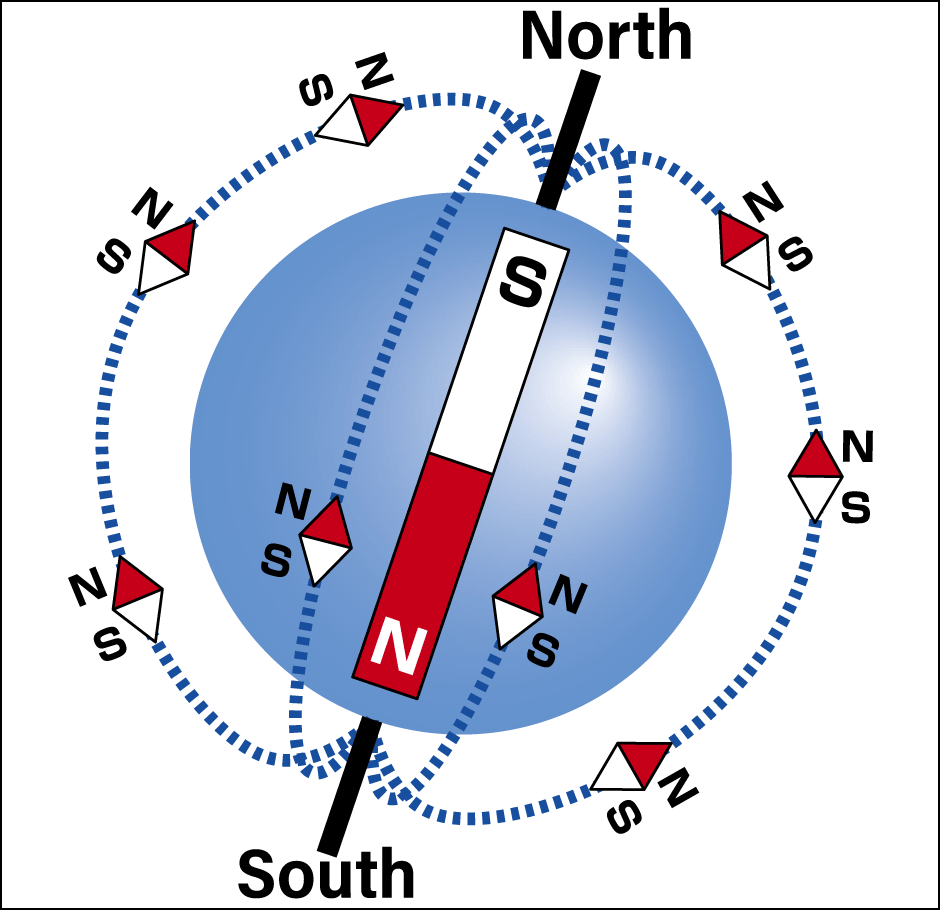
Figure 1. Geomagnetic fieldThe Earth is a giant magnet around which magnetic lines of force flow (the geomagnetic field). Instead of being parallel to the Earth’s surface, these magnetic lines of force tilt toward the North and South Poles. Animals may use these inclinations to determine their direction and location.
When you consider that the Earth’s magnetic field is about 0.5 gauss, while an ordinary magnet is 1,000 gauss or more, you can see how weak it is. While we humans do not feel the geomagnetic field, the magnetic sensitivity of animals has been studied for decades.
The magnetite hypothesis, which focuses on magnetic microparticles called magnetite in the body, was considered as the most plausible theory. Simply put, this hypothesis held that the magnetite binds to nerves and acts as a bar magnet-type compass, just like an ordinary magnetic compass. However, even though we know that magnetite is also present in humans and has a number of different functions in many body tissues, the question of whether or not it works like a compass is still just a conjecture.
The new radical-pair mechanism hypothesis
In recent years, research has advanced to explain physical phenomena in the body at the microscopic level of molecules, atoms, and electrons based on quantum theory. Research topics include energy transport in plant photosynthesis, and DNA mutations. A new hypothesis —— the radical-pair mechanism hypothesis —— has been proposed to explain the compass function in migratory birds, and efforts to shed light on this mechanism are progressing.
It was a 1972 study of European robins (Erithacus rubecula) by biologists Wolfgang and Roswitha Wiltschko of Goethe University Frankfurt, in Germany, that first sparked discussion of the geomagnetic compass in migratory birds. Some European robins head north when they migrate in spring. In this study, the researchers placed European robins exhibiting migratory restlessness in funnel-shaped cages and observed while they kept the robins in a state in which information about the surrounding environment was blocked. As the robins scratched the cage in the direction in which they wanted to fly, the researchers analyzed paper on which the birds’ movements had been transcribed and found that they showed a directional preference for north in spring. When the geomagnetic field was then masked and north created by means of an artificial magnetic field, the birds showed a preference for that direction, demonstrating that they use the geomagnetic field to determine the direction.
This study was later replicated by a research team led by biologist Henrik Mouritsen of the University of Oldenburg in Germany. However, the European robins did not exhibit any behavior that indicated a migratory direction, even during the migration season. Rather than negate the results of the Wiltschkos’ experiment, Mouritsen and his team investigated the laboratory environment. They found the environmental low-level radiofrequency noise. When the researchers blocked out this noise, the European robins began to orient themselves in the correct direction, confirming that they use the geomagnetic field to determine direction.
At the same time, the results of this study suggested the possibility that even low-level radiofrequency noise could interfere with the geomagnetic compass ability of migratory birds. Mouritsen and his team sounded the alarm that interference from the electrical devices and radio waves we use in everyday life is affecting bird migration patterns, posing a problem that could even threaten the survival of migratory birds.
Numerous research teams have carried out many other experiments that have revealed things that cannot be explained by the conventional magnetite hypothesis. For instance, the geomagnetic field —— the lines of force of the Earth’s magnetic field —— do not run parallel to the ground toward north. Studies of avian behavior when the direction of the magnetic fields is reversed have shown that the geomagnetic compass of the European robin and other long-distance migratory birds is an inclination compass that detects both the direction of the magnetic lines of force and the angle they form with the Earth’s surface. Magnetite, on the other hand, is a bar magnet-type compass that indicates the north and south poles, so one would expect it to measure polarity directly, not inclination. Another fact that casts doubt on the magnetite hypothesis is the fact that if a magnetic field were too strong, birds would not be able to sense direction, and their magnetic compass would only work if the field was about the same strength as the geomagnetic field.
In this situation, the radical-pair mechanism hypothesis was proposed by a team of U.S.-based physicists from the University of Illinois at Urbana-Champaign, a team led by physicist Klaus Schulten. A radical is an atom or molecule that has a lone unpaired electron. Normally, electrons form pairs that occupy the same orbit, but the transfer of electrons due to powerful energy such as heat or light, or breaking of chemical bonds leads to the formation of radicals. When these radical molecules form pairs that are more or less side by side, they are called radical pairs.
Acquiring the properties of a magnet
At the same time, the electrons not only orbit the nucleus, but also rotate themselves; this self-rotation of the electrons is called electron spin. Electron spin has a direction and magnitude, expressed as either ↑ or ↓. Normally, when electrons are paired, each has the opposite spin direction ↑↓, which cancels out the spin. However, in the unpaired electron of a radical molecule, the spin produces a magnetic moment (a vector representing the magnitude and direction of the magnetic moment), which gives the electron the properties of a magnet (Figure 2).
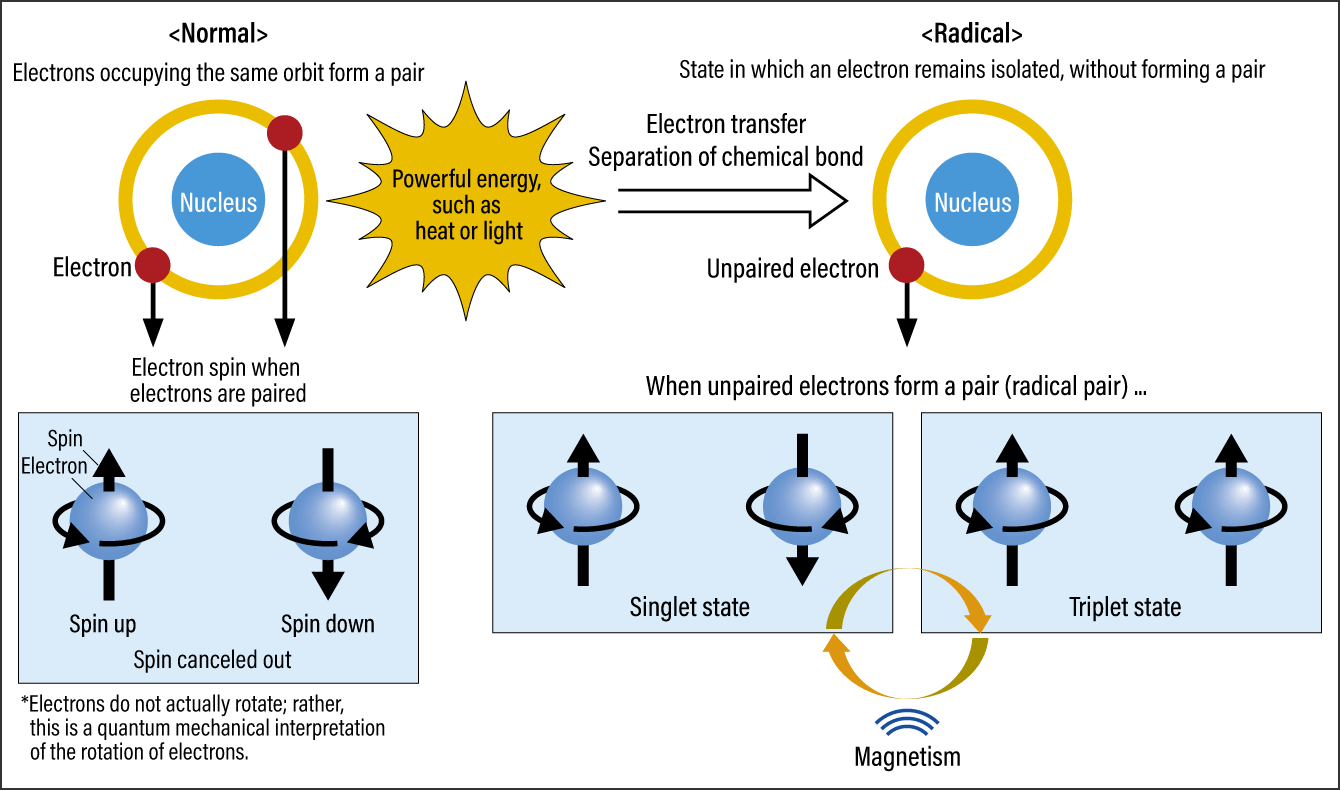
Figure 2. Radical pairs and electron spinThe self-rotation of electrons is likened to a spinning motion and is called electron spin. In the unpaired electron of a radical molecule produced by powerful energy, such as heat or light, the spin produces a magnetic moment, giving the electron the properties of a magnet. When radicals with unpaired electrons form pairs, the electron spin of both changes in a complex manner.
In radical pairs, the electron spin of each molecule is either antiparallel ↑↓ (singlet state) or parallel ↑↑ (triplet state). For example, complex changes occur in which a radical pair is initially produced in a singlet state, then changes to a triplet state before returning to a singlet state. Such changes are caused by weak magnetic fields and are known to cause changes in chemical reactions. We also know that radical pairs are produced in the initial processes of organic light-emitting diode, solar cells, and the like, as well as in photosynthesis in biological systems.
Schulten et al. suggested that the avian retina may contain a molecular system that forms radical pairs through a photochemical reaction, and that these molecules are proteins called cryptochromes. Present in plants and animals, including humans, cryptochromes are photoreceptors that absorb blue light; in animals, they are involved in the regulation of the circadian clock, while in plants they are involved in the regulation of the hypocotyl length, among other functions. Recently, scientists have also discovered that cryptochromes contain a chain of three or four molecules of an amino acid called tryptophan located close to the blue-light-absorbing flavin adenine dinucleotide, and that when blue light is absorbed, electrons are transferred between the tryptophan molecules, causing radical pairs to form. Predicting the formation of such radical pairs, Schulten et al. thought that the probability of singlet states and triplet states in them would vary with the geomagnetic field, and that this variation would act as a signal that influences the birds’ vision (Figure 3). This means that birds sense direction because, for example, north looks different —— it appears brighter or darker.
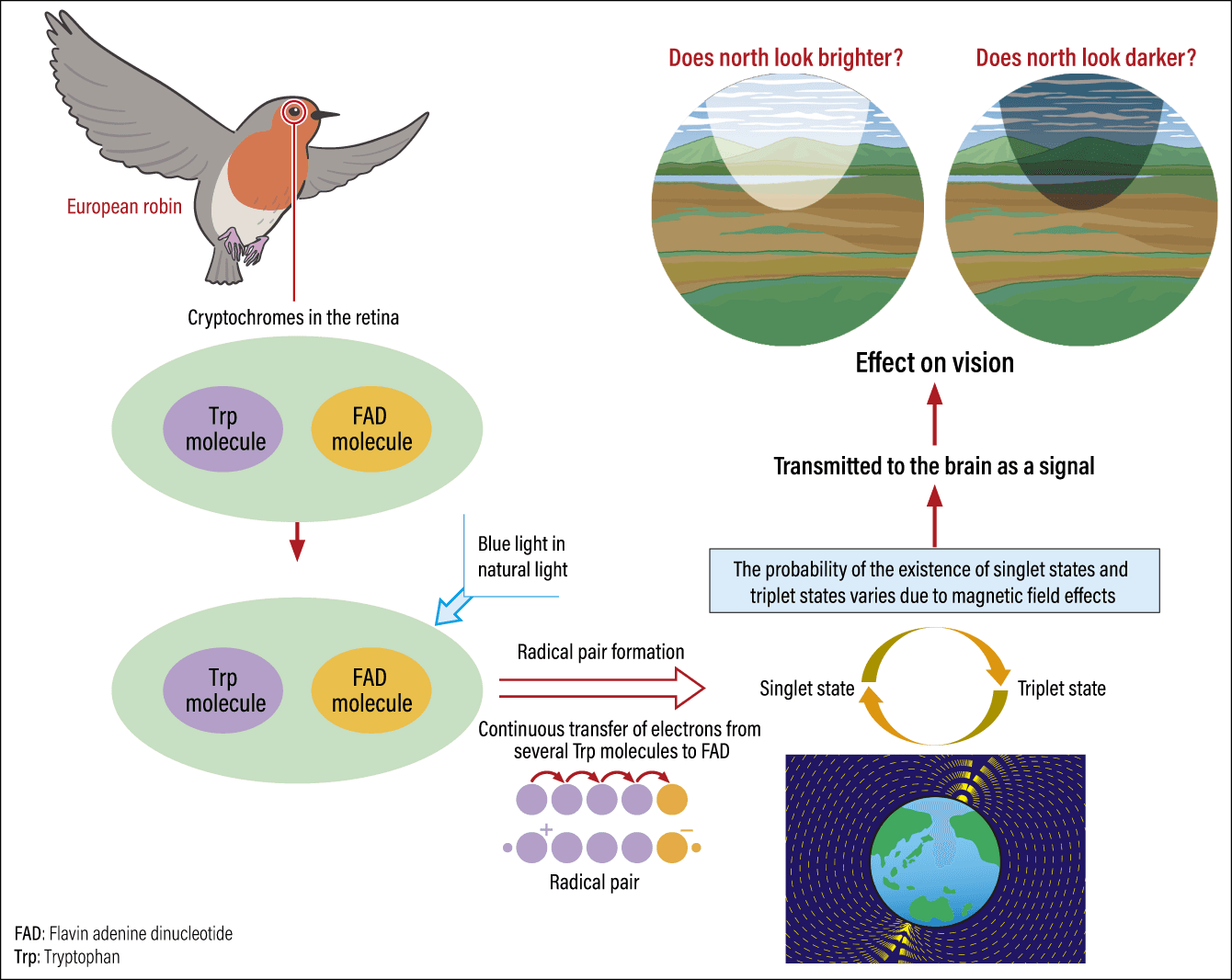
Figure 3. The navigation system of migratory birds according to the radical-pair mechanism hypothesisScientists have surmised that a molecular system in which a photochemical reaction forms radical pairs serves as a geomagnetic compass in migratory birds. The molecules involved are believed to be cryptochromes in the avian retina, and research in this area is currently progressing.
This hypothesis stunned researchers when it was published in 2000, and many expressed skepticism that radical-pair reactions could actually occur at the level of the geomagnetic field. However, in a subsequent experiment that we operated, focusing on a model compound that is physically similar to cryptochromes, we were able to confirm that the radical pairs produced by absorption of a laser beam underwent a change in chemical reactivity in response to a magnetic field at the level of the geomagnetic field, and that this response was anisotropic —— that is to say, different depending on the direction of the magnetic field —— albeit in a magnetic field of 33 Gauss, which is stronger than the geomagnetic field. Although this study did not focus on cryptochromes themselves, these results caused a great stir. Later, in an experiment we conducted with cryptochromes derived from thale cress (Arabidopsis thaliana), we were able to confirm that there are magnetic field effects in a magnetic field of about 10 Gauss (about 20 times the level of the geomagnetic field) at low temperatures. Thanks to advances in genome editing technology, we are now able to conduct experiments using synthetic birds’ cryptochromes.
Optimized through evolution
A study that has recently been attracted attention is one reported in 2021 by a team led by Peter Hore of the University of Oxford and the aforementioned Henrik Mouritsen. To date, six cryptochromes have been identified in the eyes of migratory birds; this study synthesized cryptochrome 4a (Cry4a) from the European robin. Scientists already knew that Cry4a readily forms radical pairs due to its molecular structure, and that Cry4a levels are higher in the spring and autumn migration seasons than in winter and summer, when birds do not migrate. In addition, Cry4a has a chain of four tryptophan molecules, which is thought to increase the magnetic sensitivity of migratory birds.
When these samples were measured using laser beam technology, the research team found that radical pairs had formed in the third and fourth molecules of the four-molecule tryptophan chain, and that these molecules were magnetically sensitive. As part of the study, the team also synthesized the Cry4a from the non-migratory bird species chicken (Gallus gallus) and pigeon (Columba livia), and compared them with the Cry4a from the European robin. They reported that hardly any magnetic field effects were observed in the pigeon and chicken Cry4a samples, while the European robin sample showed the highest magnetic sensitivity. Based on these results, the research team suggests that Cry4a in European robins may have been optimized through evolution for navigation. Unfortunately, this study did not shed light on whether cryptochromes in European robins show magnetic field effects at the level of the geomagnetic field, nor whether there is anisotropy. However, it is probably fair to say that the results of this study show an increased likelihood of magnetic field effects in cryptochrome reactions in migratory birds.
Advances in spectroscopy and other observation technologies will be crucial in order to verify geomagnetic magnetic field effects and anisotropy at the level of single molecules such as cryptochromes. Conventional spectroscopy methods have a number of problems, including the fact that exposing samples to laser beams and other forms of energy degrades them, and the fact that it is difficult to capture the reaction being studied, such as spin dynamics, where the change occurs within a microsecond or nanosecond as a result of excessively powerful light. We are working to develop new spectroscopic methods and devices to enable highly sensitive, stable observation of the effects of small magnetic fields. In addition, in collaboration with other laboratories at our university, we are working on the synthesis and purification of the cryptochromes and molecular dynamics calculation for investigating the mobility of the biomolecular systems.
While biological research on actual birds is, of course, important, we are keen to contribute to such research through an approach based on quantum mechanics and molecular science. It is very interesting to see that efforts to shed light on the quantum mechanical phenomena of biomolecules are progressing as a result of the fact that research aimed at unraveling the mysteries of migratory birds is flourishing. In my view, radical pairs are very important and ubiquitous things —— present all the time, everywhere. I hope that bringing to light the mechanism behind the geomagnetic compass of migratory birds will not only reveal the effects of magnetism on humans and other organisms, but also explain all kinds of biological phenomena.