Blood vessels are a very important part of the body. However, there are many aspects of their structure and function on which light has yet to be shed. In clinical settings, it is reportedly not uncommon to see blood vessels in inexplicable states. Developed to unravel such mysteries, high-resolution 3D imaging technology has succeeded in visualizing the vascular network in elaborate detail. As a result, the vascular network that scientists could hitherto only imagine has been revealed to be unexpectedly diverse. These new discoveries are likely to lead to treatments for various diseases.
Special Feature 1 – Understanding Blood Vessels Unexpectedly diverse —— The manifold mysteries of blood vessel structure and function
composition by Rie Iizuka
Blood vessels are organs essential to maintaining life, as they serve as the pipeline for the efficient delivery of oxygen from the lungs and nutrients from the intestines throughout the body.
The network of blood vessels reaches virtually every part of the human body. The total length of blood vessels in the body is a matter for debate, with some citing the figure of 100,000 km and others 6,000 km, but either way, blood vessels form the same kinds of patterns, with hardly any differences between individuals.
Capillaries are the place where nutrients and oxygen are exchanged
Blood pumped out of the heart flows from the arteries into the capillaries and then returns via the veins. Arteries are surrounded by smooth muscle cells that pulse strongly in the direction of the capillaries. Veins also have smooth muscle cells, but the muscle structure is weaker than in the case of arteries. As veins have valves, the blood cannot flow backwards, but it is the movement of the body that enables the blood to flow back to the heart.
The blood traveling through the body’s blood vessels is delivered to the capillaries without delay, with virtually no leakage from the arteries and veins. Capillaries are the place where cells in each part of the body exchange nutrients and oxygen. Capillaries have lots of holes through which blood seeps, enabling substances to be exchanged appropriately. The size of the holes in capillaries differs from one organ to another. For example, in the kidneys, the exchange of electrolytes and waste products takes place constantly, so the holes are larger than those in other organs. However, in the case of tissue such as muscle or skin, which only transfer substances when needed, the holes in capillaries are comparatively small.
The structure of blood vessels themselves is more or less uniform, from major arteries right down to capillaries. They consist of three layers: the tunica intima, tunica media, and tunica externa. The most important is the innermost layer, the tunica intima, which may be more precisely described as a layer of vascular endothelial cells adjacent to the lumen. Although it consists of only a thin layer of cells, it would be no exaggeration to say that this layer is the main body of the blood vessel.
The main function of the tunica intima is to establish the boundaries of the blood vessel. The smooth flow of blood through the blood vessels is the normal state of affairs while the coagulation of blood within the blood vessels is abnormal. However, there needs to be a means of swiftly guarding against blood leakage if a blood vessel tears. In other words, from the blood’s perspective, it needs to know where it can allow the platelets that cause blood to coagulate to work. The endothelial cells represent that demarcation line.
Blood vessels grow steadily from the fetal stage right through to adulthood. After reaching adulthood, capillaries and other blood vessels hardly ever fluctuate between regression and growth.
However, when healing after an injury or if the flow of blood has stopped due to a myocardial infarction or similar event, new blood vessels are generated from the surrounding blood vessels in order to supply oxygen to the relevant organ. This process of new blood vessels forming from existing blood vessels is called angiogenesis.
Angiogenesis is a major topic
Angiogenesis is a major topic in vascular research. One reason for this could be that angiogenesis is thought likely to be useful in treating a variety of diseases. For example, cancer cells infiltrate surrounding blood vessels and gradually generate tumor blood vessels, which serve as a supply route for nutrients. Developing the ability to cut off those supply routes is likely to lead to treatments for cancer. To achieve this, we must learn about the mechanism of angiogenesis.
The fundamental players in angiogenesis are oxygen and vascular endothelial growth factor (VEGF). Tissue in a hypoxic state for some reason produces VEGF in response to a signal from hypoxia inducible factor (HIF), then, VEGF induces the blood vessels to form new branche s—— this is thought to be the general process involved in angiogenesis.
However, as I have often seen blood vessels in states that cannot be explained by this process alone in clinical settings, I began to feel a powerful need to observe blood vessels in greater detail. The study of biology starts with looking at things. To learn about blood vessels, it is vital to observe their actual state within a living body, as far as possible. Conventional methods only allowed us to look at cross-sectional views of blood vessels, which meant we ultimately were not gaining a picture of their true nature.
To obtain an overview of the vascular network at a glance, we first developed high-resolution 3D imaging technology. This showed us the unexpected diversity of each organ’s vascular network, which we were hitherto able only to imagine (Figure 1). There had previously been a tendency to regard new angiogenesis mechanisms observed in the liver and muscles as anomalies if they were not seen in other organs. However, careful observation revealed that there is abundant diversity in blood vessels, just as cells differ from one organ to another.
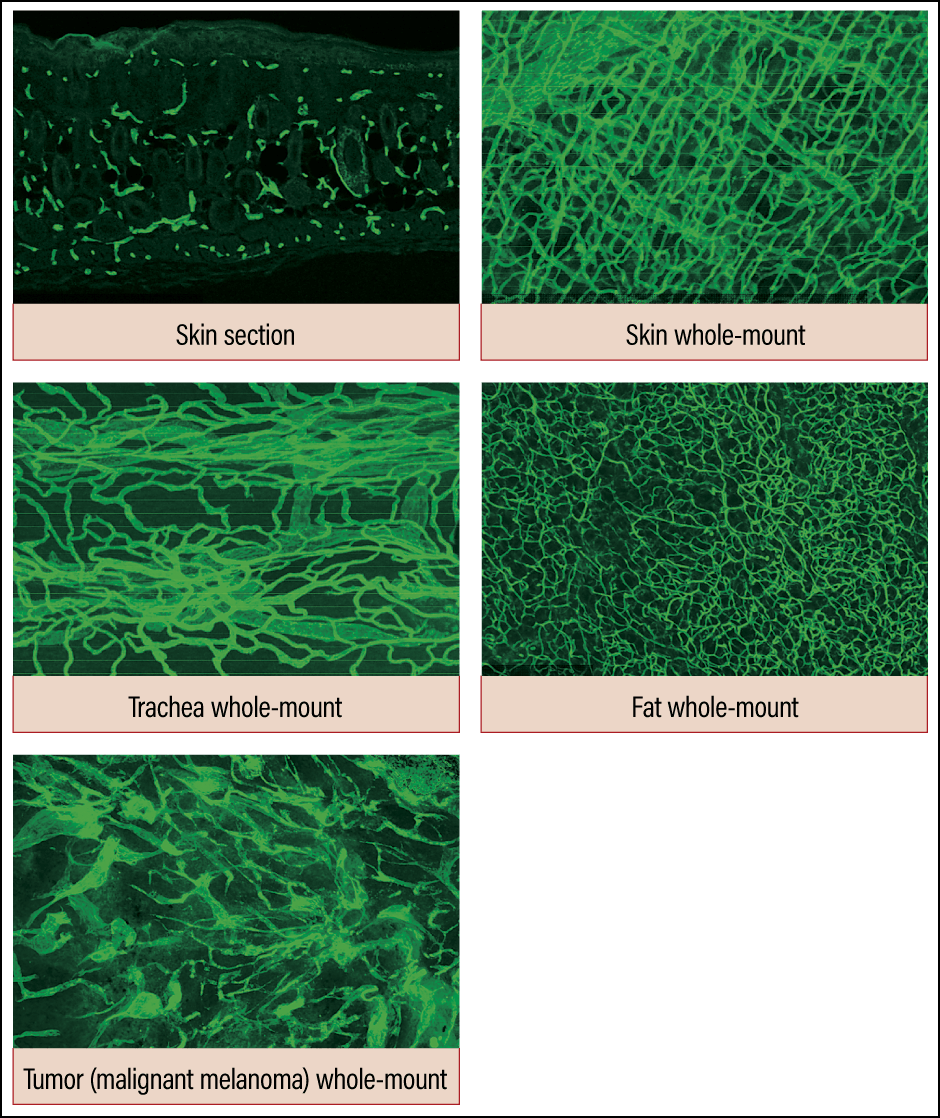
Figure 1. Diversity of vascular networksUsing the conventional method, it was only possible to observe blood vessels in a piecemeal way, as shown in the skin section image. However, high-resolution 3D imaging demonstrated that the vascular networks we had thought to be identical actually have quite different patterns in each organ.
We believe we will be able to gain a deeper understanding of angiogenesis by starting from the understanding that angiogenesis mechanisms are diverse and then identifying points in common and differences.
We recently achieved a world first by establishing a high-precision imaging technology for teeth and using that technology to shed light on the mechanism via which teeth are hardened.
Conventional diagrams illustrating dental blood vessels depicted sectional views of teeth, showing the soft tissue called dental pulp in the middle, with nerves and blood vessels running through it in a loop. However, when we observed tooth vasculature in practice, our imaging showed that blood vessels extend toward the outer surface of the dental pulp, and capillaries perforate cells called odontoblasts, which play the central role in tooth formation and hardening (Figure 2).
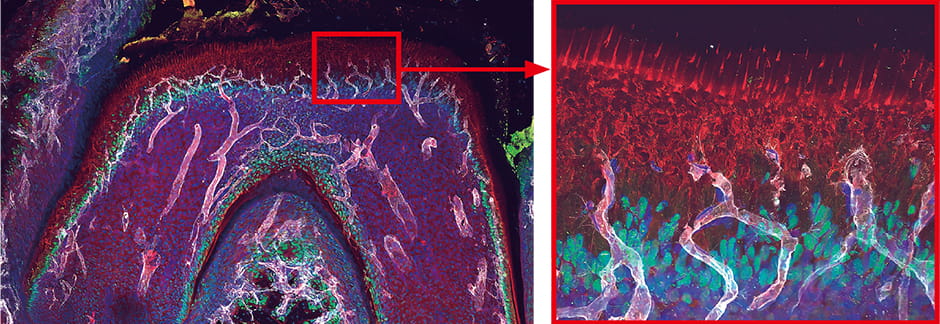
White: blood vessels; green: odontoblast nuclei; red: odontoblast processes
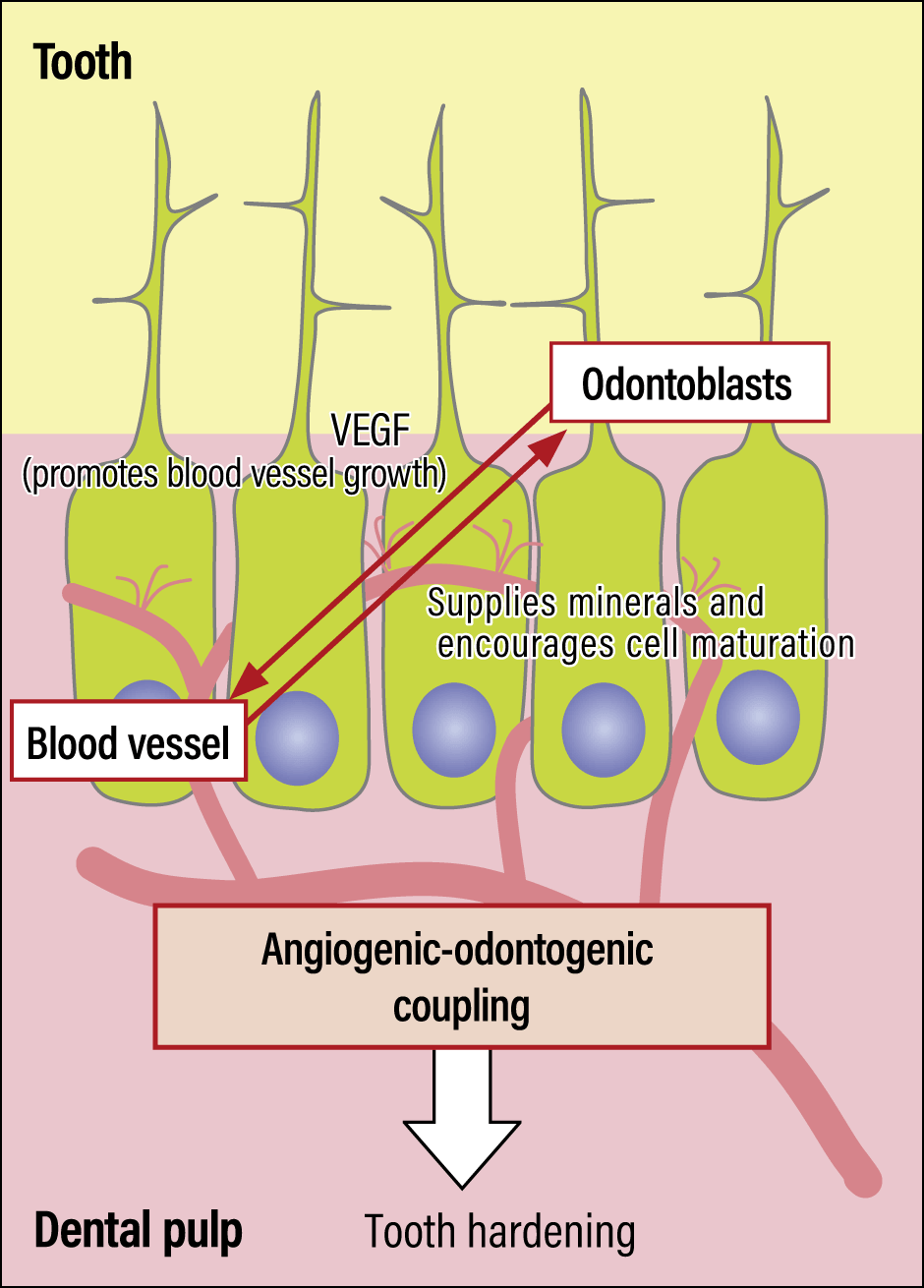
Figure 2. Blood vessels in a toothBlood vessels wrap themselves around the odontoblasts, promoting odontoblast maturation and supplying phosphorus and other minerals required for hardening.
Teeth harden as soon as they emerge from the gums, in a process called eruption. We observed an interaction based on close contact between odontoblasts and blood vessels that served to harden teeth at the time of eruption, with odontoblasts promoting blood vessel growth, and capillaries supplying odontoblasts with the elements required to harden teeth, namely phosphorus and calcium.
In fact, tooth hardening does not occur in mice that have had the capillary cell group in the center of their teeth removed. In other words, the interaction between these blood vessel cell groups and odontoblasts is thought to be an essential element of the process of tooth hardening.
It had been thought that the mechanism of interaction in teeth was the same as that between the osteoblasts involved in bone formation and capillaries. However, the molecules supplied by bone capillaries that play the central role in bone production do not emerge at all in tooth growth and hardening. Whereas in teeth, odontoblasts generate VEGF directly, in bones it is chondrocytes —— cartilage cells —— rather than osteoblasts that produce VEGF. Thus, we found that teeth and bones differ subtly in terms of the molecules produced and their interactions. Even in tissue that appears similar, such as teeth and bones, the mechanisms of blood vessel formation and the ways in which blood vessels promote the production of molecules differ.
The mechanisms and structures of blood vessels in the retina and brain differ
We have also reported that the retina and brain differ despite their apparent similarities. The retina is part of the brain and has been described as a detached force of the brain. Just like the brain, the retina is a mass of nerve cells, and it is thought to be an outgrowth of the brain formed in the process of cerebral development. The blood vessels in the brain have a mechanism called the blood-brain barrier, which severely restricts the passage of substances in either direction, and the retinal blood vessels have a similar mechanism called the blood-retinal barrier. Thus, the retina and the brain closely resemble each other both embryologically and anatomically, so it was believed that the mechanism of retinal blood vessel formation was the same as that involved in the creation of blood vessels in the brain. Consequently, it was even said that research focused on the retina, which can be observed because of its location at the back of the eye, equated to research into the brain. However, using sophisticated imaging, our team found that the retina had a different mechanism from the brain and thus the structure of its blood vessels also differed.
Both the retina and the brain are surrounded by numerous blood vessels, but the retina has a distinctive structure, in that thick blood vessels are only formed in the layer of nerve fibers found in the innermost layer of the retina, with hardly any extending into the photoreceptor cells. Along with many other researchers, I had assumed that was just the way it was, but when we actually visualized the expression of retinal VEGF receptors, we observed that the receptors believed to be expressed only in the blood vessels were, in fact, also expressed in abundance in the neurons of the retina.
We thought endocytosis might be at work here. Endocytosis is a mechanism by which cells bring in substances outside the cell by engulfing them in a vesicle, thereby enabling pathogens and the like to be broken down and digested. When we observed this process using our imaging technology, we confirmed that, as expected, retinal neurons progressively engulf VEGF (Figure 3). As VEGF is the molecule required for angiogenesis, it cannot be expressed once it has been engulfed by the cell and capillaries therefore do not form.
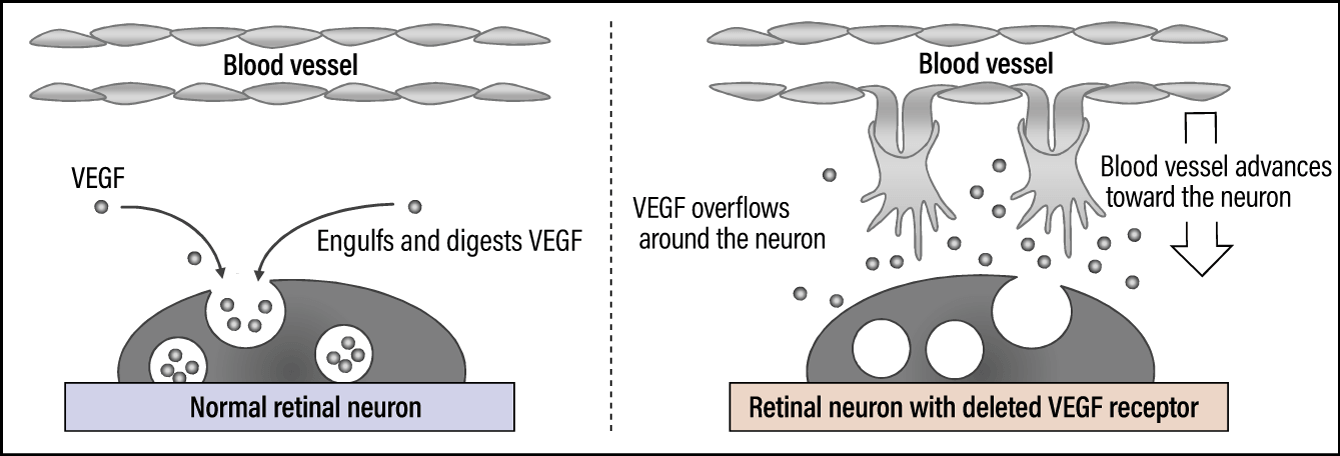
Figure 3. VEGF receptors expressed in retinal neuronsVEGF is engulfed and digested by the neuron (endocytosis), thereby limiting the advance of blood vessels.
This mechanism is thought to be related to the embryogenesis and maturation of the retina from the fetal stage.
The retina has hyaloid vessels, which are blood vessels peculiar to the fetal stage, but at a certain point, these hyaloid vessels begin to regress. The retinal blood vessels then start to radiate out from the center. It is thought that the engulfing of VEGF by neurons regulates the timing of hyaloid vessel regression.
Organs with a system of avascularity
The vitreous cavity is the central space in which the lens is located. During the fetal stage, VEGF seeps out of the neurons to promote blood vessel formation and cultivates the hyaloid vessels. However, as it would be inconvenient if those blood vessels were visible in the field of view once the infant’s eyes open after birth, the hyaloid vessels need to be made to regress without delay, so the neurons engulf the VEGF previously emitted.
This is a phenomenon specific to the retina. The brain and other parts of the central nervous system have a mechanism to ensure that thick blood vessels do not encroach on them and hinder neural activity. Thinking it would be highly interesting if the brain had the same mechanism, we conducted several experiments, but we found no evidence of a phenomenon in the brain involving neurons engulfing VEGF.
As stated above, blood vessels run throughout the body, but a number of organs are known to have a system of avascularity preventing angiogenesis like that seen in the retina. The three main avascular tissues in humans are the corneas, cartilage, and intervertebral disks. In the case of the cornea, having blood vessels would be inconvenient, as the red of the blood flowing through them would impinge on the field of view. Accordingly, instead of blood, transparent aqueous humor flows behind the cornea, providing it with oxygen and nutrients. The significance of the avascularity in cartilage and intervertebral disks has not been ascertained. However, we believe that these localized mechanisms inhibiting the growth of blood vessels are the very essence of vascular network diversity and that learning more about these mechanisms will provide us with a deeper understanding of blood vessels.
From my clinical experience, I learned that the most important thing when it comes to blood vessels is properly maintaining the hierarchy of arteries, capillaries, and veins, in order to ensure a high-quality pipeline capable of maintaining an efficient flow of blood, which carries oxygen and nutrients. I feel that the artificial formation of blood vessels that function properly is quite difficult. Some treatments for pressure ulcers (bedsores) aim to increase blood vessels, but unfortunately, even increasing the number of capillaries will not be effective if the blood vessels are weak and lack the ability to transport oxygen. In the case of pressure ulcers, creating good blood vessels from within the body by regularly changing the patient’s position and ensuring they maintained a good nutritional status ultimately led to successful treatments.
Normal blood vessels cannot be created even in cancer cells, which proliferate by infiltrating surrounding blood vessels. While blood vessels are integrated into tumors, the blood vessels formed are chaotic, and the kind of blood vessels naturally found in our bodies and formed during our development phase are not formed in cancer cells.
It is widely known that clinical testing of VEGF gene therapy to treat arteriosclerosis obliterans (ASO) has been carried out. ASO is a disease in which the progressive hardening of arteries in the legs causes the blood vessels to narrow and become blocked, preventing the maintenance of adequate blood flow and ultimately causing necrosis in the legs. VEGF gene delivery was considered as a treatment, but although it resulted in angiogenesis, the blood vessels formed were not normal, making it totally unsuitable for treating the condition and the idea came to nothing.
The sophistication of human tissue is impressive. Even though we can increase the flow of blood, increasing the number of functioning blood vessels remains beyond the limits of human knowledge, I feel. This is precisely why basic research aimed at discovering more about blood vessels is so interesting.