Cellular immunotherapy is showing promise as a new form of treatment that inhibits the various functions of cancer cells that enable them to evade the human immune system. In particular, thanks to improvements aided by the latest genome editing technology, CAR-T/TCR-T cell therapy is attracting attention as a treatment offering a decisive advantage against refractory cancer. However, as the cellular drugs covered by insurance are manufactured overseas, they have an extremely high price tag, making their widespread use among cancer patients impractical. Scientists say that the development of Japan’s own new generic technologies and license protection that do not rely on overseas intellectual property will need to be facilitated at the level of national government.
Special Feature 1 – The Truth about Genome Editing Hopes and challenges regarding the evolving field of adoptive CAR-T/TCR-T immunotherapy for cancer
composition by Toshiko Mogi
New forms of therapy are attracting attention as a trump card for cancer patients for whom the conventional treatments of surgery, anticancer drugs, and radiation therapy are expected to be ineffective. They are cellular immunotherapy approaches called chimeric antigen receptor T cell (CAR-T cell) therapy and T-cell receptor-engineered T cell (TCR-T cell) therapy.
Both involve attacking the cancer cells by modifying the patient’s own immune cells in the form of T cells, thereby strengthening the patient’s immune system.
One drug covered by Japanese insurance costs ¥33.49 million
CAR-T cell therapy is already available: tisagenlecleucel (Kymriah) received pharmaceutical approval in the U.S. in 2017, and two years later set a new record for the most expensive drug to be covered by insurance in Japan, with a price tag of ¥33.49 million. As a result, a growing number of patients are experiencing long-term remission from relapsed or refractory blood cancers against which conventional treatment methods are not fully effective.
Today, a number of other CAR-T therapies are also covered by insurance: lisocabtagene maraleucel (Breyanzi), axicabtagene ciloleucel (Yescarta), idecabtagene vicleucel (Abecma), and ciltacabtagene autoleucel (Carvykti). All of these therapies are treatments for cancers of the blood (Table).
Generic name | Brand name | Applicable diseases |
Tisagenlecleucel | Kymriah | Relapsed or refractory CD19+ B-cell acute lymphoblastic leukemia, follicular lymphoma, and diffuse large B-cell lymphoma |
Lisocabtagene maraleucel | Breyanzi | Relapsed or refractory follicular lymphoma, diffuse large B-cell lymphoma, primary mediastinal large B-cell lymphoma, high-grade B-cell lymphoma, and indolent B-cell non-Hodgkin lymphoma |
Axicabtagene ciloleucel | Yescarta | Relapsed or refractory diffuse large B-cell lymphoma, indolent follicular lymphoma, primary mediastinal large B-cell lymphoma, high-grade B-cell lymphoma |
Idecabtagene vicleucel | Abecma | Relapsed or refractory multiple myeloma |
Ciltacabtagene autoleucel | Carvykti |
TCR-T cell therapy, meanwhile, became available in the U.S. in August 2024, when afamitresgene autoleucel (Tecelra) was approved as a treatment for synovial sarcoma in adults. This marked the first time that TCR-T cell therapy had been approved as a cancer treatment. However, it has not been approved in Japan.
The following describes the use of genome editing technology in cancer treatment, with a focus on CAR-T cell and TCR-T cell therapies (Figure 1).
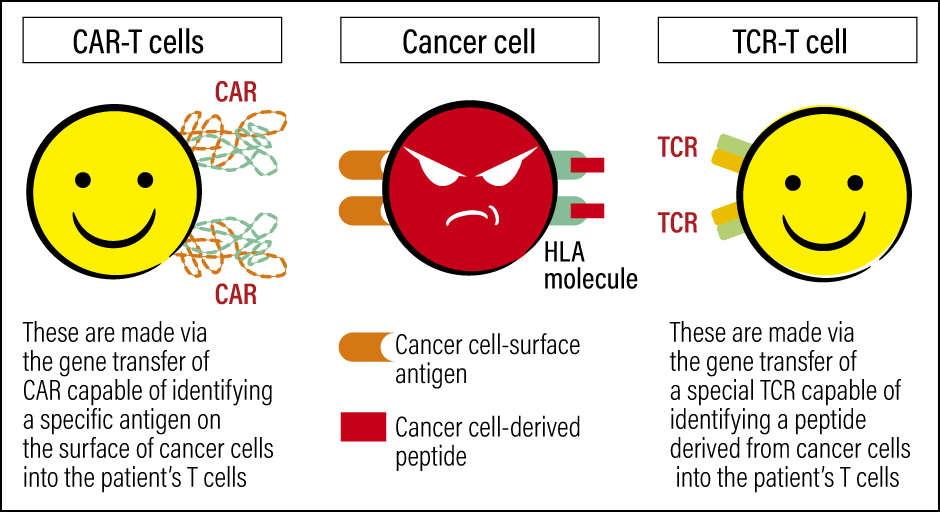
Figure 1. CAR-T cells and TCR-T cellsBoth cells strengthen the patient’s immunity and attack cancer cells, but their targets differ. CAR-T cells target antigens on the surface of cancer cells, whereas TCR-T cells target antigens specific to the patient’s cancer.
Cancers that are hard to cure or prone to recurrence are called refractory cancers. Given that some cancers can be fully cured, why are others refractory? Let us start by touching on the attack and defense mechanisms of immunity and cancer.
As most readers are well aware, our bodies are equipped with an immune system, which attacks and removes any foreign particles it finds. The central role in our immune system is played by immune cells called lymphocytes, which are categorized into T cells and B cells, and natural killer (NK) cells. Among our lymphocytes, T cells play a particularly important role, serving as a kind of control tower by directing the immune cells’ response. The T in their name is taken from the thymus, which is the gland where they are produced.
The mechanism works as follows. On the surface of T cells are found T-cell receptors (TCR), which recognize foreign particles in the body via human leucocyte antigens (HLA). Cancer cells produce various abnormal proteins; fragmented versions of these proteins (peptides) are presented by HLA molecules on the surface of cancer cells. One could describe them as cancer cell markers (cancer antigens). When immune cells find these cancer antigens, T cells use TCR to identify them as foreign particles that should be eliminated, and the attack on the cancer cells begins.
However, cancer cells have a property that enables them to hide the cancer antigens that mark them out, in order to avoid being identified as foreign particles and being attacked by the immune system. We also know that cancer triggers the abnormal proliferation of suppressor cells, which weakens the effectiveness of T cells and other immune cells.
Cancer cell identification functions and attack functions
Our immune system, on the other hand, is also equipped with a mechanism that applies the brakes to the attack, in order to ensure that our own normal cells are not damaged by an excessive immune response. Some cancer cells are able to exploit this mechanism to bring the T cell attack to a halt.
To date, there have been significant advances in technologies for the development of antibody drugs that use immunoglobulin (antibodies) to attack cancer cells. This sparked the idea of using antibodies instead of TCR to distinguish specific cancer cells from other cells. Scientists then produced a gene that combined the cancer cell identification functions of some antibodies with the cancer cell attack functions of others, so that the attack function would be switched on when cancer was identified. This was followed by the development of a cellular drug (a medication produced by manufacturing cells like a drug for administration to a patient to treat a disease) that can be inserted into the patient’s T cells to treat cancer. These are CAR-T cells. Pronounced “car-tee,” the CAR stands for chimeric antigen receptor, while the T refers to T cells.
Another treatment method taps into the innate functions of TCRs, which serve as a kind of antenna for identifying foreign particles: it involves finding TCRs capable of identifying a specific cancer antigen, and then re-inserting them into the patient’s own T cells, to produce cancer-specific T cells. This is called TCR-T cell therapy. It was actually in 2006 that a research team led by Dr. Steven A. Rosenberg of the U.S. National Cancer Institute reported the results of a clinical study that showed TCR-T cell therapy to be effective in patients with melanoma.
CAR-T cells achieve effects by identifying the common antigen projecting from the surface of specific cancer cells. TCR-T cells, on the other hand, are thought to be capable of turning antigens specific to the patient’s cancer (neoantigens) into targets. TCR-T cell therapy is therefore expected to be an effective new treatment for solid cancers, which express a particularly large number of neoantigens, and research and development is progressing in this area.
Both CAR-T cell therapy and TCR-T cell therapy could be described as bespoke therapies tailored to each individual patient’s condition. This is because both employ the following process in order to treat each patient (Figure 2).
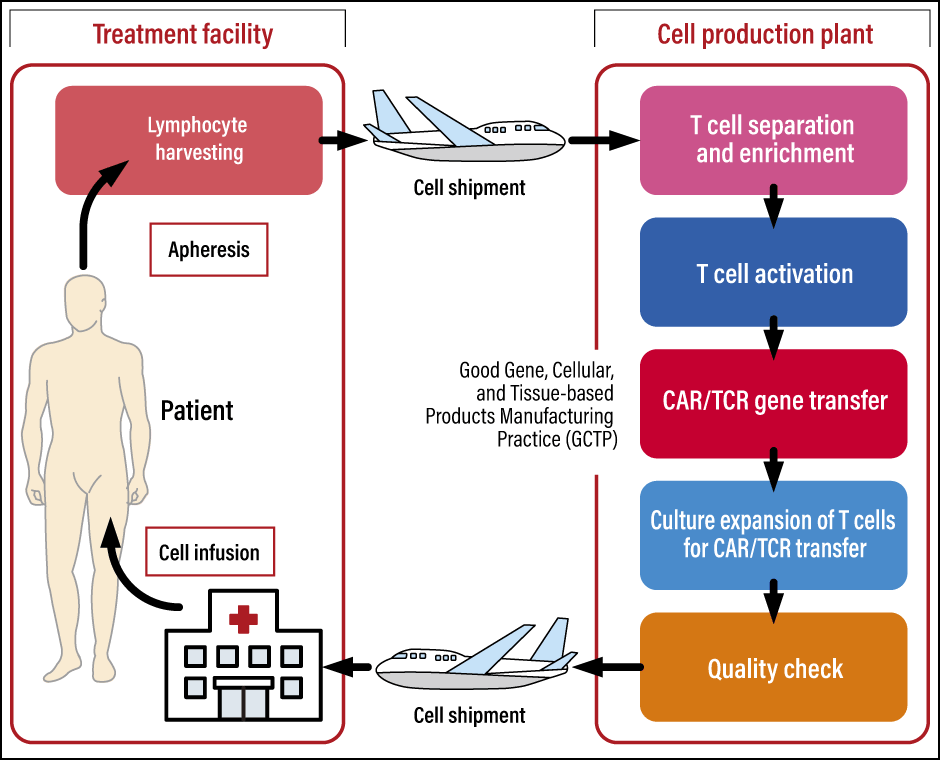
Figure 2. The process of manufacturing genetically modified T cellsBoth CAR-T and TCR-T involve harvesting the patient’s lymphocytes at the treatment facility, producing genetically modified T cells at a cell production plant, and shipping the modified cells back to the treatment facility where the patient is waiting.
❬ 1: Collection of blood components from the patient (apheresis) ❭
The patient’s own lymphocytes are collected and sent to the cell production facility.
❬ 2: Production of CAR-T/TCR-T cells ❭
T cells are selected from the harvested lymphocytes. A gene vector is produced to introduce the CAR-T/TCR-T cells, then gene transfer technology is used to insert the vector into the aforementioned T cells to modify them, thereby producing the CAR-T/TCR-T cells. Culture expansion is carried out in order to produce the required number of CAR-T/TCR-T cells.
❬ 3: Administration of CAR-T/TCR-T cells ❭
Lymphodepleting chemotherapy is carried out to prepare the patient before starting the treatment. The objective is to facilitate the proliferation of the administered CAR-T/TCR-T cells in the body by reducing the number of lymphocytes in the body beforehand. The CAR-T/TCR-T cells are administered by means of infusion or injection.
After administration, the CAR-T/TCR-T cells bind to cancer cells that have expressed antigens in the patient’s body and begin to attack them. If this attack on the cancer cells is successful, the patient will go into remission.
The world’s first treatment using CAR-T cells derived from a third party
While CAR-T cell therapy is a minimally invasive treatment compared with hematopoietic stem cell transplantation and the like, it still presents challenges that need to be solved, as it can have such distinctive side-effects as cytokine release syndrome and late-onset combined immunodeficiency. In Japan’s case, it takes a long time before the actual administration can begin, as the cells have to be sent overseas, because there are no domestic manufacturing plants. During this period, the patient’s condition may deteriorate and there is a possibility that the CAR-T cells will not be able to be administered.
In addition —— and this is something that could be said of regenerative medicine as a whole —— as the cells are produced using cells harvested from the patient, the effects will differ from one individual to another. In the case of refractory cancers, the cells derived from the patient have often been depleted by the treatment the patient has already received. If the patient’s T cells have been weakened, the worst-case scenario is that the production of CAR-T cells will not be possible.
Modified T cells derived from a third party were devised as a means of resolving this issue. The idea behind this approach is that if CAR-T cells are produced from lymphocytes donated by a healthy donor beforehand and then cryopreserved, they can be used as soon as treatment is required. Accordingly, in 2015, a team from University College London became the first in the world to produce CAR-T cells derived from a third party’s genome-edited cells, using them to treat two pediatric patients with leukemia.
Why was genome editing required? T cells are equipped with a function that enables them to use TCRs to distinguish themselves from another person’s T cells. If the donor’s T cells are administered to the patient as they are, the TCRs expressed on the modified T cells derived from the donor will actually attack the patient’s healthy organs. This is called graft versus host disease (GVHD).
To avoid this toxic reaction occurring in the patient’s body, the donor’s TCR gene has to be knocked out when producing the third-party-derived CAR-T cells. Accordingly, it is cut out using genome-edited scissors so that GVHD cannot occur.
The world’s first treatment using CAR-T cells derived from a third party succeeded in reducing the number of leukemia cells. At the time, it was unclear whether CAR-T cell therapy alone would be sufficient to achieve remission, so both patients subsequently received hematopoietic stem cell transplants from healthy donors. Following this report, research focused on producing CAR-T cells derived from third parties flourished worldwide.
The price of tisagenlecleucel —— the first CAR-T cell drug derived from a patient’s own cells to be approved —— has now been revised to around ¥32.65 million in Japan, which is cheaper than when insurance coverage was first granted. As no third-party-derived CAR-T cells produced by genome editing have yet been brought to market, we do not know how much they will cost. The price of such drugs when brought to market is a matter of great concern.
Developmental research aimed at increasing the safety of both CAR-T cell and TCR-T cell therapies and achieving better effects from them is progressing in Japan and overseas. In Japan in particular, the development of domestically produced novel T-cell drugs that employ technology originating within Japan is eagerly awaited.
This is because, as described above, Japan lacks the manufacturing sites required for CAR-T cell therapy and there is also little domestic production technology, so licensing foreign technology and sending cells overseas and back again requires a great deal of time and money. If domestic technology can be used to manufacture the cells within Japan, it will likely reduce both the cost and time involved.
While prices have been amended, all CAR-T cell drugs cost in excess of ¥32 million. As the Japanese government has put in place such systems as a high-cost medical expense benefit, the cost burden on the patient differs according to their age and income, among other factors. The applicability conditions are restricted, so fewer patients can make use of it than in the case of other diseases, which means that the finances of Japan’s health insurance system are not yet being placed under pressure. However, it would be fair to say that curbing skyrocketing drug prices is also a social issue, from the perspective of maintaining the finances of Japan’s universal health insurance system based on mutual aid.
Pursuing the development and licensing of technology unique to Japan
We at Hiroshima University have been working on the development of TCR-T cell therapy. Based on the cancer antigen NY-ESO-1, we aimed to use Platinum TALENs —— a genome editing tool developed at Hiroshima University —— to develop a TCR-T cell therapy that demonstrates outstanding safety and effectiveness (Figure 3).
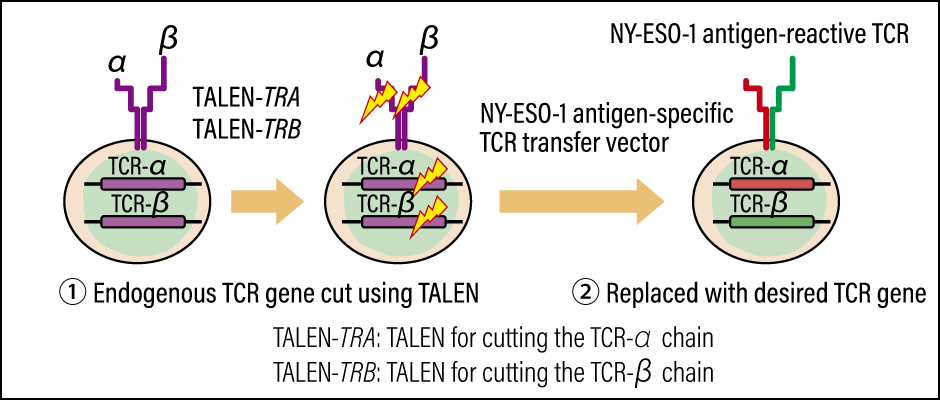
Figure 3. Production of NY-ESO-1 antigen-specific genome-edited T cellsThe concept involves producing a modified gene cassette with enhanced attack capabilities that targets NY-ESO-1, and accurately replacing the patient’s TCR gene.
In healthy tissue, the expression of NY-ESO-1 is restricted to the testes alone. However, this cancer antigen’s expression has been observed in some blood cancers and in numerous solid cancers, including cancers of the esophagus, lung, breast, ovary, prostate gland, and bladder, as well as melanoma.
While there are high hopes for its application in treatments for various cancers, some scientists have pointed out that TCR-T cell therapy poses an issue in terms of interference with endogenous TCRs. Specifically, if competition occurs between the TCRs that were originally present in the patient’s body (endogenous TCRs) and the transgenic TCRs, there is a possibility that mispairing could occur between them.
To avoid this situation, we first deactivated the patient’s TCR gene with the aid of genome editing technology using Platinum TALENs, and then transferred the cancer antigen-specific TCR gene.
Conventional CAR-T and TCR-T approaches used a viral vector to transfer genes to T cells. However, this method posed a problem, in that the genes were transferred to random positions on the genome. While the probability is low, there is a possibility that the transfer of the vector to an unexpected location could activate cancer-causing genes called oncogenes , or cause other hazardous mutations. Accordingly, we decided to include in our development topic the establishment of a technology for accurately transferring the NY-ESO-1 antigen-specific TCR transfer vector to the site of the TCR gene.
Using all these technologies, we aimed both to establish a key technology for producing a human cell-processed drug in the form of genome-edited TCR-T cells capable of recognizing NY-ESO-1, and to develop a technology to guarantee the safety of cell drugs produced using genome editing. Put simply, our goal was to use genome editing technology to make a genetically modified cassette that would not interfere with endogenous TCRs, and to create a T-cell drug that would slot neatly into the patient’s TCR gene.
The Japan Agency for Medical Research and Development (AMED) selected this project for inclusion in the Cyclic Innovation for Clinical Empowerment (CiCLE) program in 2019, and our work had been proceeding since then. Unfortunately, however, we ended up taking the decision to halt our developmental research in 2024.
A diverse array of technologies are essential to drug discovery. The rights to these technologies are protected by licenses. It is an undeniable fact that this situation is reflected in the high prices charged for drugs. The obstacle standing in the way of our research was overseas licenses. The sum involved vastly exceeded our expectations and it was this, coupled with the headwind of a weak yen, which forced us to abandon our project. It was a heartbreaking experience, given that we had been achieving positive results.
Genome editing technology will undoubtedly be the key to the development of next-generation medical care. I am painfully aware that, in order to enable patients to use the therapies and drugs created as a result with peace of mind and without putting the finances of our medical insurance system under pressure, two things will be required: the development of Japan’s own unique new key technologies that do not rely on overseas intellectual property, and an intellectual property strategy at the national government level that protects the licenses to those technologies.