Blood vessels play a part in the transport of nutrients and oxygen to cells and tissues, while vascular endothelial cells themselves preserve the body’s homeostasis by secreting substances that contribute to tissue maintenance in the process of their differentiation and proliferation (blood vessel formation). Cancer cells are able to multiply because they generate blood vessels in cells that did not originally have blood vessels and therefore become able to take in nutrients. Vascular abnormalities are said to be closely connected to infectious diseases such as COVID-19, along with a variety of other diseases. Shedding light on blood vessel formation will undoubtedly become a new approach to treating disease.
Special Feature 1 – Understanding Blood Vessels Will shedding light on blood vessel formation open the door to new treatments for disease?
composition by Rie Iizuka
illustration by Koji Kominato
One of the key themes in vascular research is blood vessel formation. Research in this area focuses on the mechanism via which blood vessels are formed.
Vasculogenesis produces blood vessels and blood all in one go
There are two types of blood vessel formation. One is angiogenesis, which is a system in which the body’s existing blood vessels develop sprouts that grow to form new blood vessels. Likened to the formation of buds on trees, it is sometimes called sprouting. Once an individual has reached maturity, angiogenesis occurs regularly in the endometrium in response to the estrous cycle, as well as when the body is trying to repair an injury. It almost never occurs in any other situation.
However, in the case of cancer, angiogenesis occurs in cells that originally had no blood vessels and branches gradually form from surrounding blood vessels, taking in nutrients and causing the repeated abnormal proliferation of cancer cells. Accordingly, research in this field is thriving, as there are hopes that unraveling the mechanism of angiogenesis could lead to cancer treatments if we can suppress tumor blood vessels.
The other route of blood vessel formation is vasculogenesis, in which blood vessels are formed when angioblasts become differentiated during the fetal stage.
Near the fetus is found a membrane called the yolk sac, which provides nutrients. Figure 1 shows the process of blood vessel formation in this part. The numerous dots are called blood islands, which are formed when as many as 100 cells derived from the mesoderm clump together. These islands gradually begin to join up, like beads on a necklace, becoming a single string. The cells on the outside differentiate into vascular endothelial cells, while those on the inside differentiate into blood. Vasculogenesis is a very efficient system for producing blood vessels and blood all in one go during the early stages of fetal development; it is believed that it almost never occurs after the body reaches maturity.
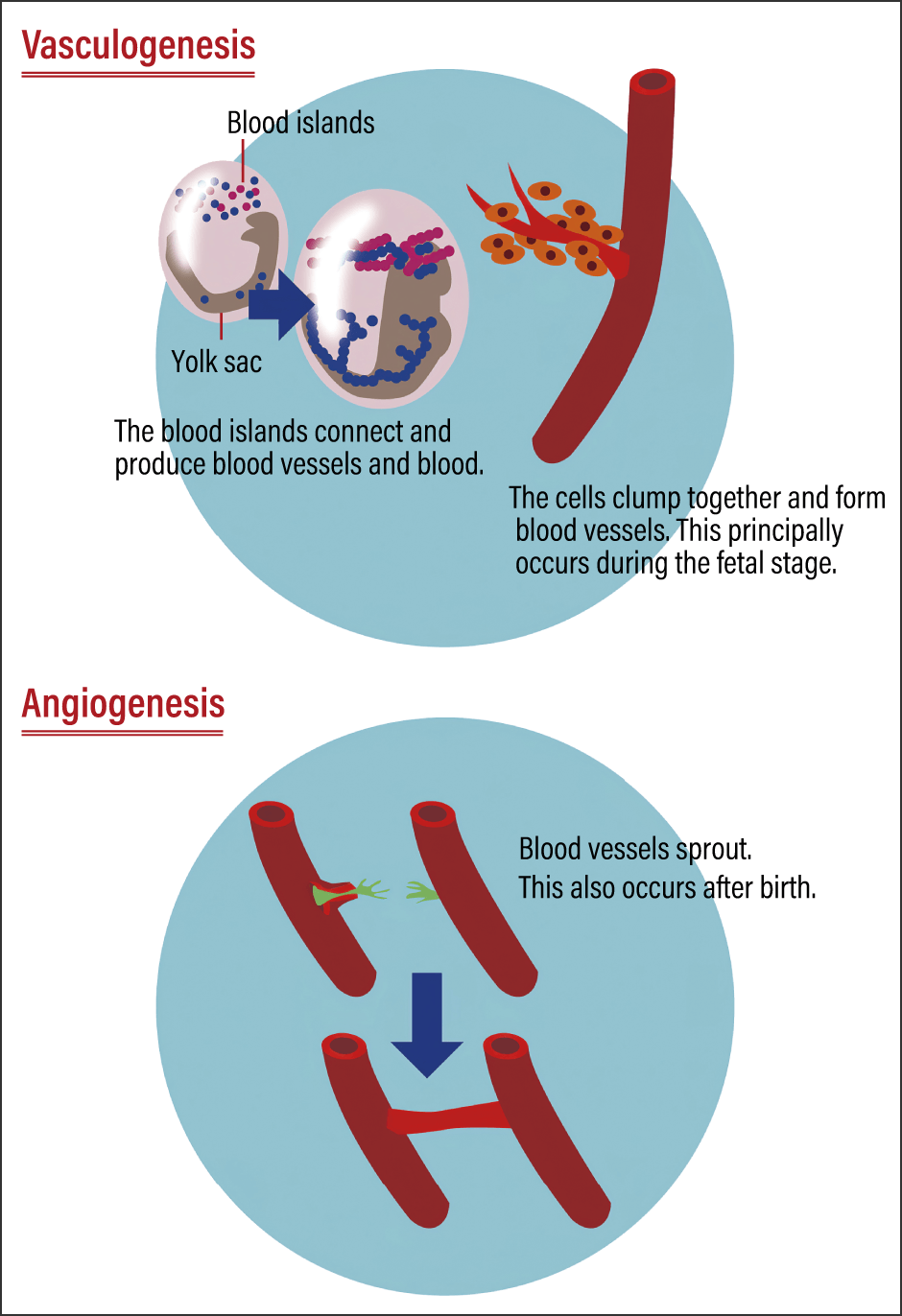
Figure 1. The two types of blood vessel formationIn the case of vasculogenesis, blood islands are formed as a result of the differentiation of cells derived from the mesoderm. These blood islands connect to each other like a string, and blood vessels and blood are then produced. This is a different mechanism from angiogenesis, in which blood vessels form from existing blood vessels.
Whether as a result of vasculogenesis or angiogenesis, a neatly laid out configuration of blood vessels resulting from those mechanisms and a vascular network of capillaries and the like are then formed. This is called vascular remodeling.
Figure 2 shows vascular remodeling in mouse skin. First, thick blood vessels are formed in haphazard fashion. These then serve as the starting point for the formation of networks of capillaries via angiogenesis. The blood vessels are all jumbled up to begin with, but arteries and veins (the yellow and blue lines in Figure 2) then gradually start to become aligned in parallel and change into functionally mature blood vessels. This process is believed to be more or less the same in humans and in mice.
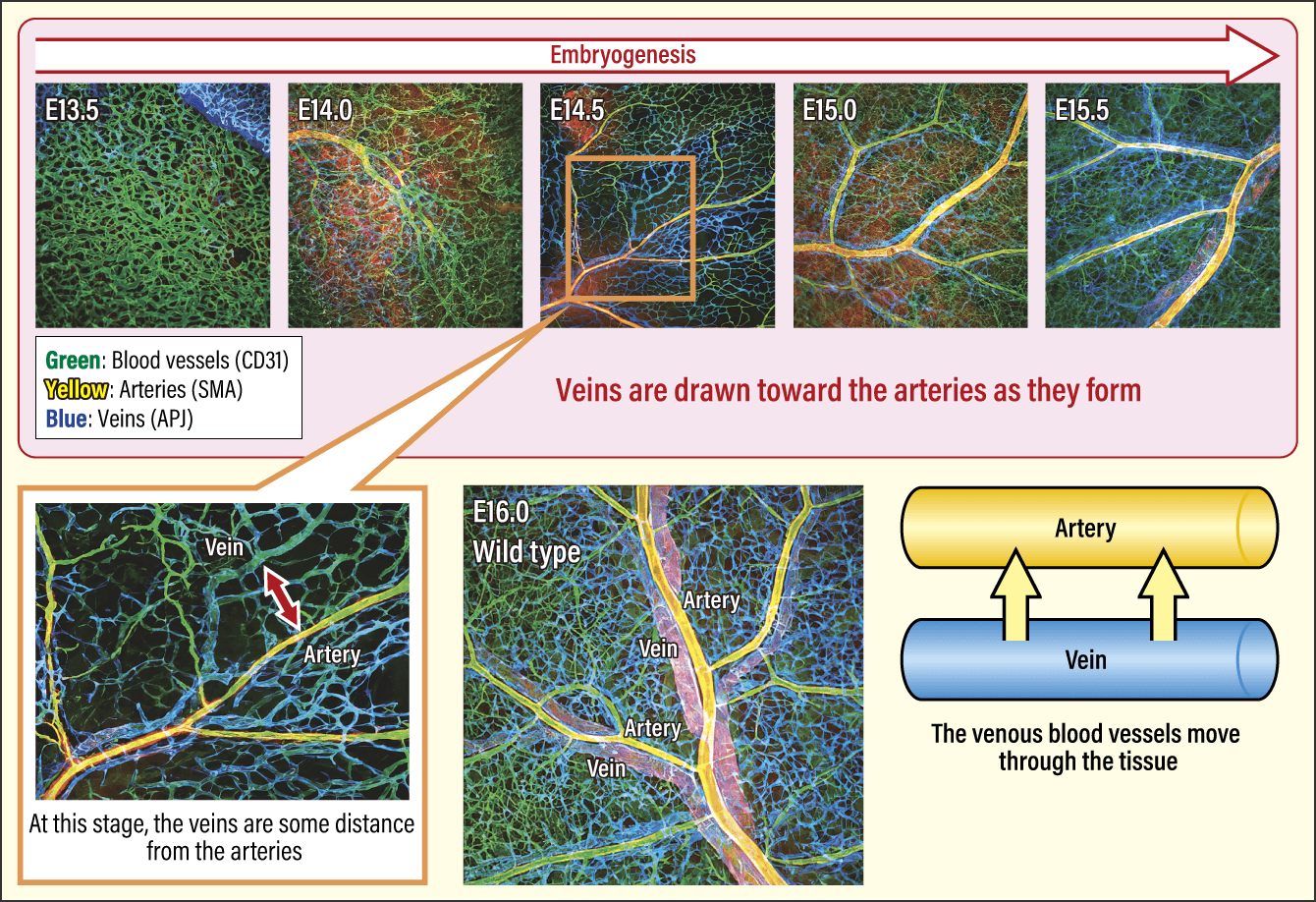
Figure 2. Vascular remodeling in miceThe fetal stage in mice lasts about 20 days. Blood vessels gradually form at around 7.5-8.5 days after fertilization, and the flow of blood can be observed once the heart starts moving at 9.5 days. The structure of the vascular network is still immature even at 13.5 days after fertilization. The arteries and veins gradually get closer to each other and finally become aligned in parallel.
The Belgian anatomist Andreas Vesalius was the first in the world to sketch all the blood vessels of the human body. Published in 1543, his classic anatomy book De Humani Corporis Fabrica Libri Septem (On the Fabric of the Human Body in Seven Books) depicts the structure of the body’s arteries and veins neatly aligned in parallel. However, we still have not unraveled the question of why blood vessels develop a parallel structure. Accordingly, our team started by investigating the timing at which this parallel alignment of arteries and veins develops.
Substances that play a key role in angiogenesis
Looking at the skin of a mouse embryo at 13.5 days after fertilization (E13.5) in Figure 2, while the body has grown to some extent by this stage, the structure of the blood vessels is still chaotic. But by 14.5 days (E14.5), the veins seem to have been drawn closer to the arteries and gradually become aligned in parallel.
I focused on a bioactive oligopeptide called apelin, thinking that between arteries and veins there might be some kind of interactional system like a ligand and its receptor that causes the blood vessels to become aligned in parallel from their originally disorganized state. Apelin is expressed in various tissues and is known to play an important role in angiogenesis.
When I looked at the expression of apelin during the parallel alignment of blood vessels, I found that apelin was expressed in the arteries, while the apelin receptor APJ was expressed in the veins (Figure 3). From this observation, it would appear that the peptide secreted from the artery stimulates the receptor in the vein, causing the vein to move. To verify this, we observed knockout mice in which either apelin or APJ was not expressed and found that their veins did not move, and parallel alignment of their arteries and veins did not develop (Figure 4).
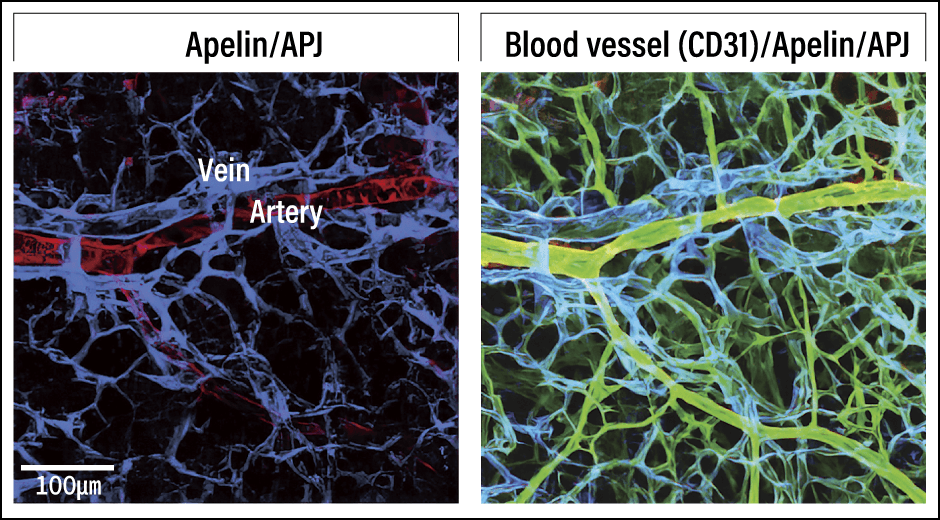
Figure 3. Arterial-venous alignment processA peptide called apelin (red) on the artery side and a receptor called APJ (blue) on the vein side are specifically expressed and the apelin acts on the APJ.
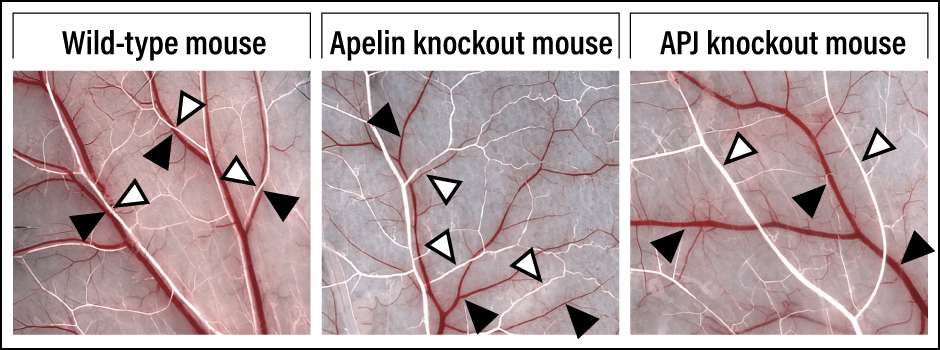
Figure 4. Differences in vascular networks according to whether or not apelin and APJ are expressedWhile the arteries (white) and veins (red) in the wild-type mouse become aligned in parallel, they remain separated in apelin and APJ knockout mice.
Our team reported this movement of blood vessels as a phenomenon called vascular strand invasion mediated by the interaction between the two.
What is profoundly interesting is that the knockout mice lead their lives in a way that does not differ at all from wild–type mice. Even though their vascular networks are in chaotic state, there is apparently no impact on their health. If that is indeed the case, why are veins and arteries aligned in parallel?
Parallel alignment of arteries and veins maintains body temperature
In fact, a 1948 article described countercurrent heat exchange when reporting that the parallel alignment of arteries and veins serves to maintain body temperature. Blood flowing to the body’s extremities when the ambient temperature is high is warmed by the ambient temperature once it reaches the extremities. When that blood returns to the body’s core, the core temperature ends up rising. This article surmises that there might be a mechanism in which the arteries and veins are aligned in parallel to transfer the heat in order to prevent this from happening.
To test that hypothesis, we placed a wild-type mouse with arteries and veins aligned in parallel and a knockout mouse with separated blood vessels in a high-temperature environment and measured changes in their body temperature. We found that the knockout mouse’s body temperature soon rose, and it developed heat stroke within a short time. Conversely, when we lowered the temperature, the knockout mouse’s core temperature fell sooner than that of the wild-type mouse. The parallel alignment of arteries and veins thus appears to maintain body temperature.
In the animal kingdom, there are homeotherms and poikilotherms. Let us look at how this relates to the parallel alignment of arteries and veins. The boundary between species that demonstrate arterial-venous alignment and those that do not lies between birds and frogs (Figure 5). From this, too, it seems that parallel alignment of arteries and veins maintains body temperature.
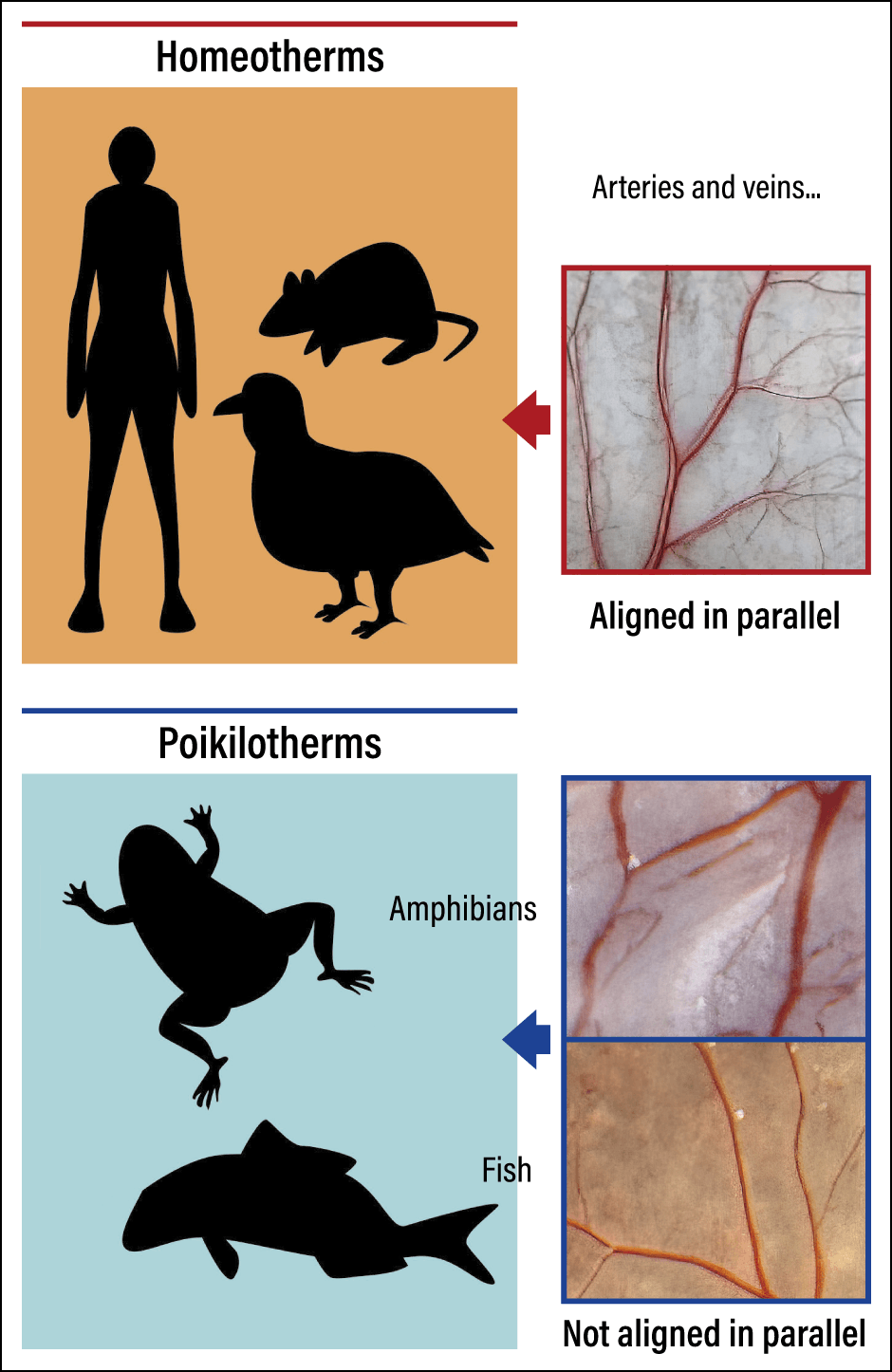
Figure 5. Differences in the vascular networks between different types of faunaIn humans, mice, and birds, which are homeotherms, the arteries and veins are aligned in parallel, whereas in frogs and fish, which are poikilotherms, they are not.
Apelin is one of several bioactive substances called angiocrine factors that are secreted by vascular endothelial cells. As well as functioning as pipelines carrying nutrients and oxygen, blood vessels have an important function as organs that secrete hormone-like substances.
Angiocrine factors were classically believed to be molecules like apelin secreted from vascular endothelial cells that played a part in blood vessel formation. However, it has recently begun to become clear that they also serve to maintain homeostasis in nearby tissues other than blood vessels.
Blood vessel states differ depending on the organ. Tissue-specific vascular endothelial cells produce tissue-specific angiocrine factors that maintain homeostasis in the relevant tissue.
For example, the hepatic sinusoid produces a molecule called hepatocyte growth factor, which is believed to have a functional role in the proliferation and repair of hepatocytes. It is also known that the vascular niche microenvironment contains angiocrine factors working within.
Hematopoietic stem cells normally lie dormant next to the blood vessels of the bone marrow; when needed, they differentiate and proliferate near the blood vessels to produce blood cells. The signal that they are needed comes from the angiocrine factors secreted by vascular endothelial cells, which are believed to control the dormancy, differentiation, and proliferation of hematopoietic stem cells.
It was believed that blood vessels were located within a few micrometers of tissue to supply it with oxygen, but their presence close to each type of tissue is also efficient from the perspective of producing angiocrine factors to issue instructions to that tissue.
Vascular abnormalities also observed in COVID-19 cases
Thus, the role of blood vessels as a kind of control tower has suddenly come under the spotlight. But how does this mechanism function in the event of illness? This raises the question of whether it is the control function of blood vessels malfunctioning that allows disease to develop.
Naturally, one thinks of cancer in this context. If an individual develops leukemia, the hematopoietic stem cells differentiate in abnormal ways. The vascular endothelial cells in the bone marrow appropriately control the timing of the proliferation of hematopoietic stem cells and the location of their differentiation. However, in the case of leukemia, it is thought that the blood vessels malfunction and the vascular endothelial cells issue what one might call adverse orders. Regulating the condition of blood vessels in mice with leukemia has actually resulted in curbing the progression of the disease.
Taking this observation into account means that we need a new perspective on treating cancer. While the blood vessels have malfunctioned, that does not mean they are cancerous. However, it is thought that some kind of abnormal stimulus is transferred from cancer cells to vascular endothelial cells, causing the latter to produce substances that issue an adverse order. I call this the angiocrine switch.
For example, leukemia is believed to be a type of cancer that is prone to recurrence, and the conventional treatment has been to kill the leukemia cells. However, it is conceivable that cells could become cancerous again because the blood vessels that malfunctioned at the onset of leukemia remain as they were.
Vascular abnormalities are thought to be involved not only in cancer, but also in a variety of other illnesses, including infectious diseases. Vascular abnormalities have also been observed in COVID-19 cases. It is unclear whether the vascular abnormalities occur first and cause the symptoms of the disease to worsen, or whether severe symptoms cause abnormalities in the blood vessels, but either way, vascular abnormalities are conspicuous in severe cases of COVID-19. Tests have shown a therapeutic effect resulting from the use of a drug that restores to normal the disordered production of angiocrine factors caused by COVID-19.
While the target here is to eradicate a virus causing an infectious disease, it is becoming clear that in this case, too, regulating the condition of blood vessels so that vascular endothelial cells secrete positive angiocrine factors results in the alleviation of symptoms.
We think that there might also be substances that induce the angiocrine switch in the case of diabetes and aging, so we are exploring this possibility.
While blood vessels serve as a pipeline for the smooth supply of substances, they are also organs that secrete appropriate angiocrine factors. As such, I believe maintaining vascular health will become an increasingly important concept in the future.