The extracellular environment —— that is to say, the environment outside the cell —— is composed of proteins secreted by cells and is deeply involved in cell function. The same can be said of vascular cells: it has been discovered that in arteries, which are exposed to the stresses of blood pressure and blood flow, rubberlike elastic fibers principally composed of a protein called elastin, which is secreted by vascular cells called smooth muscle cells (SMCs), are deeply involved in the development of arterial diseases such as aortic aneurysm and aortic dissection. Also underway is research into biomarkers for predicting the onset of disease using the knowledge obtained on the extracellular environment.
Special Feature 1 – Understanding Blood Vessels The proteins in the extracellular environment involved in arterial diseases
composition by Yumi Ohuchi
illustration by Rokuhisa Chino
The cells of which bodies are composed do not function on their own; rather, they keep the body in a stable state by altering their cytoskeleton (tissue within the cell that maintains the cell’s shape) and dynamics in response to changes in the microenvironment between cells, which is called the extracellular environment. It would be no exaggeration to say that the extracellular environment alters cells and controls their fate.
Protein structures secreted by cells themselves
Various factors are present in this extracellular environment, but the main component is the extracellular matrix (ECM). The ECM, which could be described as a cell’s habitat, is a structure made of collagen, proteoglycan, and other complex macromolecules secreted by the cells themselves. The cells slot neatly into the ECM, indispensable part of our bodies.
The ECM differs from one tissue to another: for example, in the case of bone, the ECM secreted by osteoblasts is calcified to produce hard tissue. In the case of blood vessels, the ECM produced by cells maintains their flexibility and strength. The fact that abnormalities in the vascular ECM are related to the pathology of cerebrovascular and cardiovascular diseases has become the focus of attention in recent years. Through research centered on the connections between vascular cells and the ECM and their responses, we are endeavoring to shed light on the pathology of the major arterial diseases, namely, aortic aneurysm and aortic dissection.
In the three–layer structure of blood vessels, the innermost layer consists of endothelial cells, which are surrounded by layers of SMCs, which in turn are covered by fibroblasts in the most outer layer. The major components of the ECM are elastic fibers produced by SMCs and collagen fibers produced by fibroblasts (Figure 1). Elastic fibers consist of a rubberlike protein called elastin, which stretches when force is applied and returns to its original state when the force is removed. The collagen in collagen fibers gives blood vessels the strength required to withstand being pulled (tensile strength).
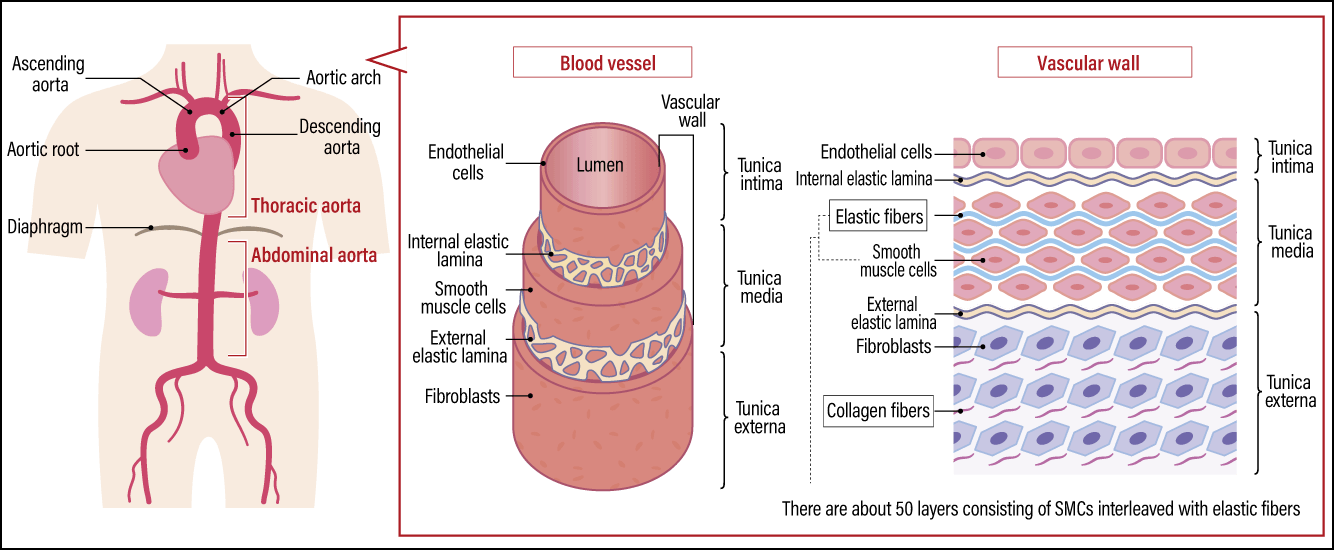
Figure 1. Vascular structure of the aortaThe aorta is a blood vessel running from the thorax to the abdomen that pushes blood throughout the body. The vascular wall of the aorta is thicker than that of other blood vessels, with a greater abundance of elastic fibers, in order to withstand mechanical stress from blood pressure and blood flow. Aortic aneurysm and aortic dissection are two major diseases that occur in this part of the body.
In arteries, the elastic fibers are particularly important and are found in greater abundance than in veins. Of the various arteries in the body, the aorta has the effect of causing blood vessels to dilate during the systolic phase and return to normal during the diastolic phase, pushing blood to every extremity of the body. Thus, the vascular wall of the aorta is constantly exposed to mechanical stresses in the form of stretch and shear stress due to blood pressure and blood flow. Consequently, elastic fibers are highly developed, and the aorta has around 50 layers of SMCs interleaved with elastic fibers in a manner reminiscent of an onion. It is often said that our blood vessels harden as we age, because the fragmentation of these elastic fibers and a decline in their number reduces elasticity, causing tensile strength from collagen fibers to increase.
Fragmentation and condensation of elastic fibers were observed
Some 20 years ago, we reported that a protein called Fibulin–5 (Fbln5) was essential for the formation of these elastic fibers. Fbln5 is one of the ECMs that had been discovered at that time and was known to be found in the human body, particularly in areas with an abundance of elastic fibers.
In this study, we generated Fbln5 knockout mice (Fbln5KO mice) and observed the course of their growth. While the Fbln5KO mice grew to adulthood, their aortas became elongated as they grew and an astonishing phenomenon occurred wherein their skin would, if pinched, stretch and spread out like a skirt (Figure 2). We also found that this phenomenon progressed as they aged. Under observation with an electron microscope, fragmentation and condensation of the elastic fibers were found. In addition, we found abnormalities in the lungs and vagina —— parts of the body that normally have an abundance of elastic fibers —— such as emphysematous lungs and pelvic organ prolapse (a condition in which one or more of the bladder, uterus, rectum, and vagina slip down from their normal position). The findings from this study formed the starting point for my current research.
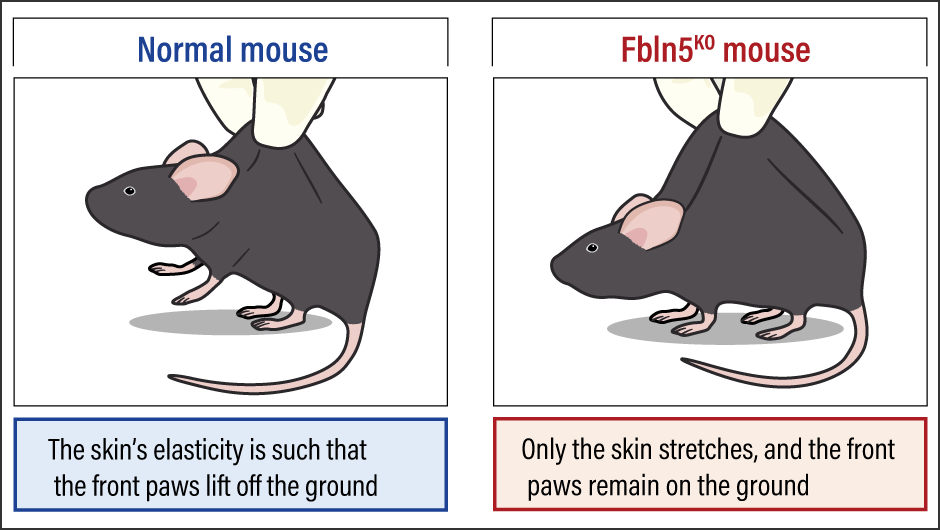
Figure 2. Fbln5 knockout mouseWhen the skin on the back of the Fbln5KO mouse was pinched, it stretched further than that of the normal mouse and spread out like a skirt.
An aortic aneurysm occurs when the aorta, which usually has a diameter of around 20 mm, enlarges to more than 1.5 times that size, forming a protuberance. It is a very dangerous condition, because it does not heal naturally and if it ruptures, the resulting major hemorrhage can be fatal. While arteriosclerosis is said to be one cause, the precise mechanism of onset is still not known. Such aneurysms are broadly classified into thoracic aortic aneurysms and abdominal aortic aneurysms, depending on their location; thoracic aortic aneurysms are believed often to be the result of a congenital abnormality in the ECM. However, in the aforementioned Fbln5KO mice, although the elastic fibers were destroyed, the mice did not develop aortic aneurysms. We then focused on Fibulin-4 (Fbln4), which is part of the same family as Fbln5.
Fbln4 is also involved in the formation of elastic fibers and collagen fibers, and Fbln4 knockout mice have been reported to die shortly after birth from such conditions as the rupture of aortic aneurysms.
To avoid causing death to newborn mice and enable our research to continue, we generated mice in which Fbln4 was knocked out specifically in vascular SMCs (Fbln4SMKO mice). Aortic aneurysms were subsequently observed in all cases three months after birth (Figure 3).
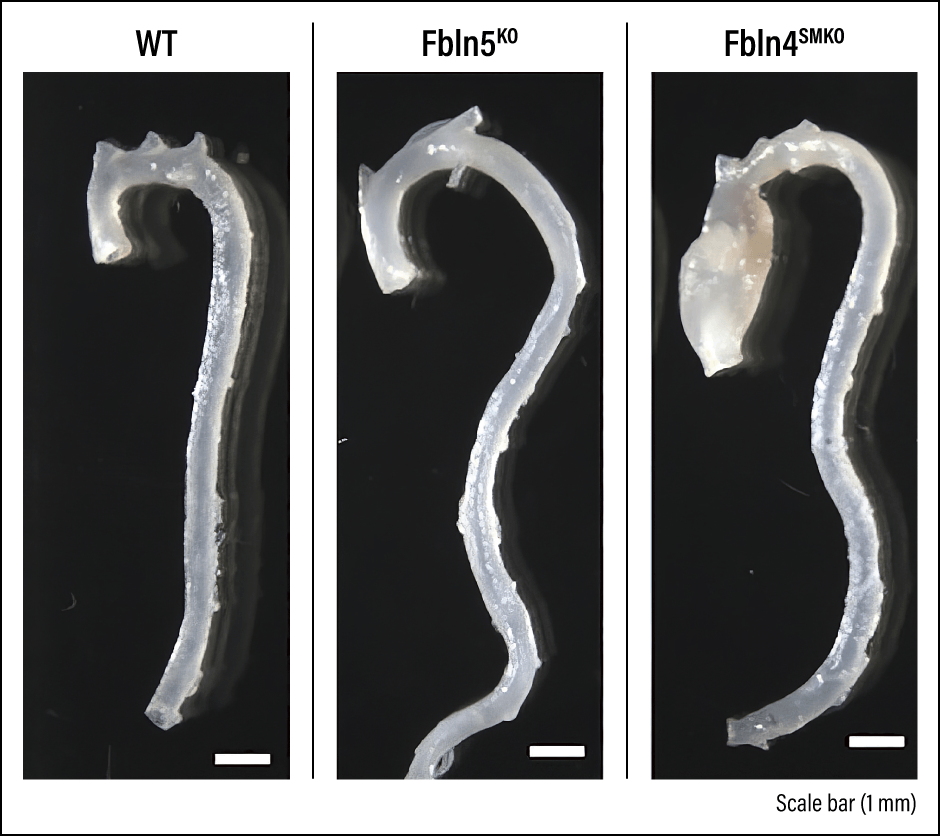
Figure 3. Comparison of ascending aortic aneurysmsFrom left to right, comparison of ascending aortas in wild type mouse (WT, no genetic modification), Fbln5KO mouse, and Fbln4SMKO aortic aneurysm mouse. The Fbln5KO mouse shows no onset of aortic aneurysm but does exhibit elongation of the ascending aorta. Both mice exhibit elongated and tortuous descending aorta.
Using an electron microscope, we then compared the Fbln5KO mice, which had not developed aortic aneurysms, with the Fbln4SMKO mice, to see whether there were any differences in the formation of the elastic fibers in their vascular walls. The elastic fibers in both had fragmented and demonstrated clear abnormalities. However, when we focused on the elastin extensions, which connect the elastic fibers to SMCs, we found that whereas the Fbln5KO mice still just about retained those bindings, they had been lost in the Fbln4SMKO mice. These results led us to believe that the severing of bindings between elastic fibers and SMCs is one cause of the onset of aortic aneurysm.
The protein overexpressed in aortic aneurysm
Allow me to explain the binding between elastic fibers and SMCs in a little more detail. Parts of the elastic fibers bind to the SMCs via a receptor called integrin on the surface of the SMC and are linked from these adhesion points (focal adhesion) to contractile fibers within the SMCs. In other words, the elastic fibers transmit mechanical stress and other external stimuli via these bindings, which are called elastin-contractile units, and the SMCs receive the signals, enabling them to respond to extracellular changes.
Furthermore, as a result of investigating what molecular changes take place in aortic aneurysms in Fbln4SMKO mice, we found overexpression of thrombospondin-1 (Thbs1) in the endothelial cells of the vascular wall and the nearby SMCs from the time of onset. Thbs1 is a protein present in various tissues as a form of ECM, but its expression is markedly lower in the blood vessels of normal adult mice.
When we knocked out Thbs1 from Fbln4SMKO mice, we were able to suppress the formation of aneurysms. In other words, our study indicated the possibility that if the binding between elastic fibers and SMCs has been lost, Thbs1 is overexpressed and affects the progression of aortic aneurysm. Additionally, we discovered that Thbs1 is secreted in response to stretch stimulus due to mechanical stress on SMCs, so it would appear that it plays an important role in the mechanism via which cells detect and respond to external forces, as well as in the transmission of those signals.
Nevertheless, there is still the outstanding question of why cells respond and Thbs1 rises, even though the bindings between elastic fibers and SMCs had been severed. We believe it causes an abnormality in the response to mechanical stress, because the SMCs cannot precisely detect stretch stimulus in the blood vessels.
Furthermore, with the cooperation of the University’s Department of Cardiovascular Surgery, we analyzed the tissue from lesions in 28 human patients with thoracic aortic aneurysm and found increased expression of Thbs1. As Thbs1 is expressed not only in mice, but also in humans, this indicated the possibility that suppressing Thbs1 might be useful in treating the aorta.
There are no effective drug therapies to treat aortic aneurysm at present. If the aneurysm is comparatively small and has a low risk of rupture, it is principally treated via management of blood pressure and other lifestyle improvements, while the main treatments for aneurysms large enough to be a high risk are vascular prothesis implantation, which involves replacing the aneurysm with an artificial blood vessel, and stent-grafting (implanting an artificial blood vessel inside the blood vessel). I want to shed further light on the pathology of this condition, in the hope it will lead to the development of therapeutic drugs targeting Thbs1.
Aside from aortic aneurysm, another arterial disease is aortic dissection. This is a condition in which the aortic medial layer tears, allowing blood to flow between one layer and another, thereby causing the vascular wall to thin and making it more prone to rupture. It is every bit as dangerous a disease as aortic aneurysm, and the mortality rate is particularly high if the dissection reaches as far as the ascending aorta, which is close to the heart. If the blood vessel has ruptured or is at risk of rupturing, vascular prothesis implantation is performed.
We are conducting a time course analysis study using an aortic dissection mouse model generated on the basis of genetic information for human disease, in order to examine what molecular changes occur in the vascular wall. Incidentally, we believe that, unlike in aortic aneurysm, the lack of Fbln4 is not directly involved in aortic dissection, and that the condition is mediated by a different mechanism.
Biomarkers using biogenic substances
Another project underway leverages the results of our research to date and involves measuring biomarkers to forecast the onset of aortic aneurysm and aortic dissection. A biomarker is a substance in the body that serves as an indicator of the presence or absence of a disease, changes in a patient’s condition, or the effectiveness of a treatment. There are various biomarkers, including prostate-specific antigen (PSA), which is used as an indicator of prostate cancer, and blood glucose level, which is an indicator of diabetes. Currently, aortic aneurysms are mostly found by chance, during medical examinations, for example, and regular ultrasound and CT examinations are required to assess the size of the aneurysm. Aortic dissections, meanwhile, occur suddenly and have a high risk of recurrence even after treatment, which also necessitates regular imaging examinations. There are no biomarkers for indicating the presence of an aneurysm or for predicting rupture or dissection.
In this project, we intend to apply Raman microspectroscopy, which uses scattered light illuminating a substance to gain information about its molecular structure. This approach for measurement and analysis of biogenic substances enables us to obtain spectral information (information in which waves such as light or signals have been broken down into their components, with the components arranged by size) and image those substances (Figure 4). As a result of using this technique for the measurement and analysis of thoracic aortic aneurysms in the aforementioned mouse models and human samples, we have found that the specific ECM components exist solely in aortic aneurysm mice and human aortic aneurysms. We believe the information on disease-specific ECM provided by Raman microspectroscopy in this way could potentially serve as a biomarker.
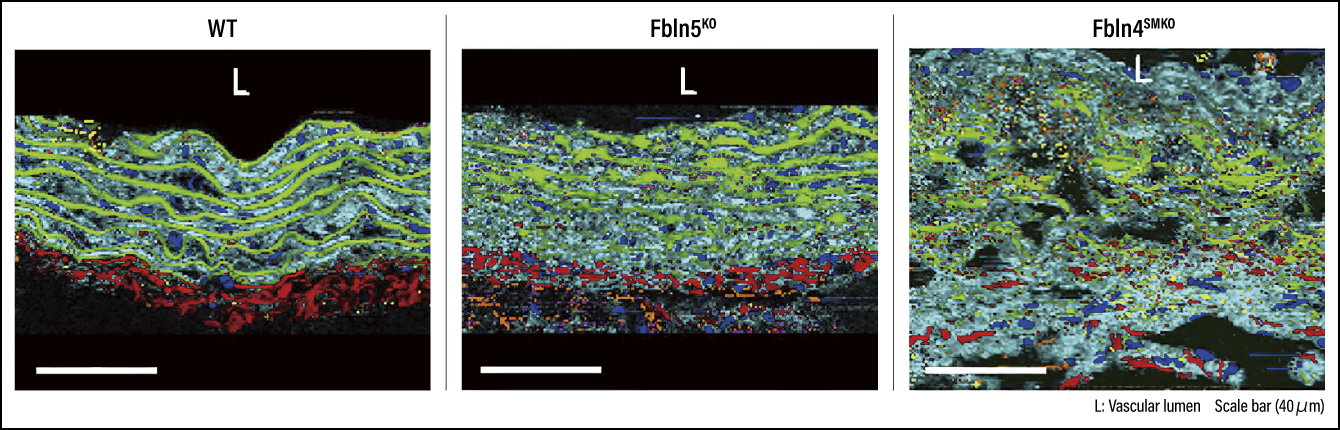
Figure 4. Label-free imaging using Raman microspectroscopyImages (Raman imaging) generated from spectral and spatial information obtained using Raman microspectroscopy. This renders biogenic substances visible, as follows: elastic fibers (green), collagen fibers (red), nuclei (blue), aggrecan (yellow), versican (magenta), lipids (orange), and residual ECM (cyan).
We are still using tissue slices as samples, but if we could develop an instrument capable of easily measuring ECMs of the vascular wall, it would likely become possible to carry out more precise and detailed evaluations than existing imaging examinations.
Research into cellular and extracellular environmental responses is becoming widespread in a variety of fields, including artificial blood vessels and other areas of bioengineering, and regenerative medicine using stem cells such as iPS cells. Studies like ours focused on blood vessels are also being carried out both within Japan and overseas, with fresh knowledge being amassed all the time. For example, several mutations have been discovered in the genes that code the structural components of elastin-contractile units, giving rise to the possibility that this could lead to the development of a genetic panel test that shows the risk of aortic aneurysm or aortic dissection. Going forward, it will likely be necessary for us to take our research to a new level by teaming up with other research groups. In addition, I hope that pursuing studies based on collaboration from the basic to the clinical level and back again will lead us to treatments and preventive medicine.