Glycans are a type of sugar, consisting of monosaccharides linked together like chains. By linking to proteins and lipids in the body, glycans not only regulate their functions, but also play an important role in cell recognition and adhesion processes, which are involved in supporting life itself. Another of their functions is determining the timing of protein metabolism, with the life span of proteins in the body or blood determined by the glycans linked to them. At the same time, glycans are drawing attention as the third most important life chain for unraveling the mysteries of biological phenomena, behind the nucleic acids and proteins responsible for the diverse evolution of living organisms.
Special Feature 1 – Focus on Sugars Playing a crucial role in metabolism, could glycans hold the key to unraveling biological phenomena?
composition by Rie Iizuka
illustration by Koji Kominato
Glycans are chains of monosaccharides such as glucose and mannose that are anywhere from several to several dozen molecules long. They are usually found linked to proteins or lipids, covering the surfaces of cells.
Responsible for the crucial role of tuning the body, glycans are thought to be a tool created by organisms to deal with events occurring within the body or cells. One familiar example is blood type. Under the ABO blood group system, an individual’s blood type is determined by that person’s glycans. This is said to be a vestige of infections suffered by our distant ancestors (Figure 1).
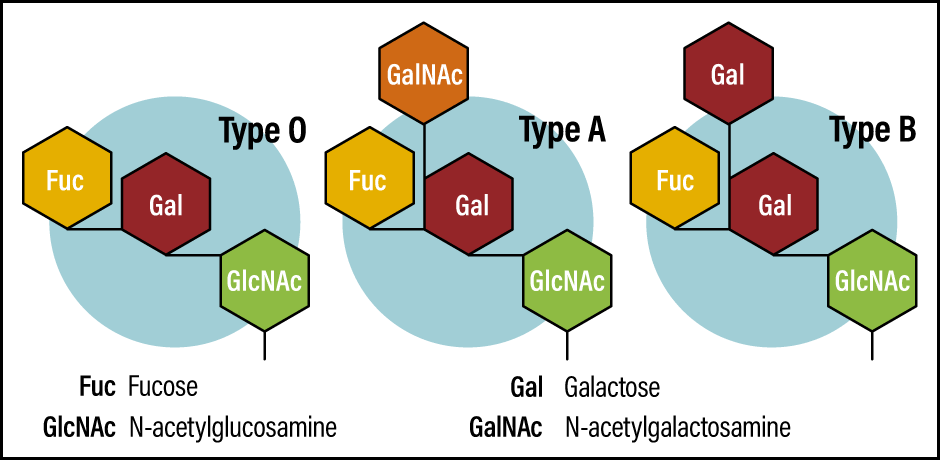
Figure 1. Blood typesBlood type is determined by glycans adhering to the surface of red blood cells. It is thought that in the case of type A, N-acetylgalactosamine (GalNAc) became linked to the glycans possessed by those with type O in order to deal with some kind of infection, and that galactose (Gal) linked to the type O glycans via a similar process is responsible for type B blood. Those with type AB blood have both these glycans.
Regulating protein and lipid functions
Let us look at the diverse roles of glycans.
As well as being involved in the physiological functions of cell recognition and adhesion, glycans are responsible for regulating the functions of the proteins and lipids to which they link (Figure 2).
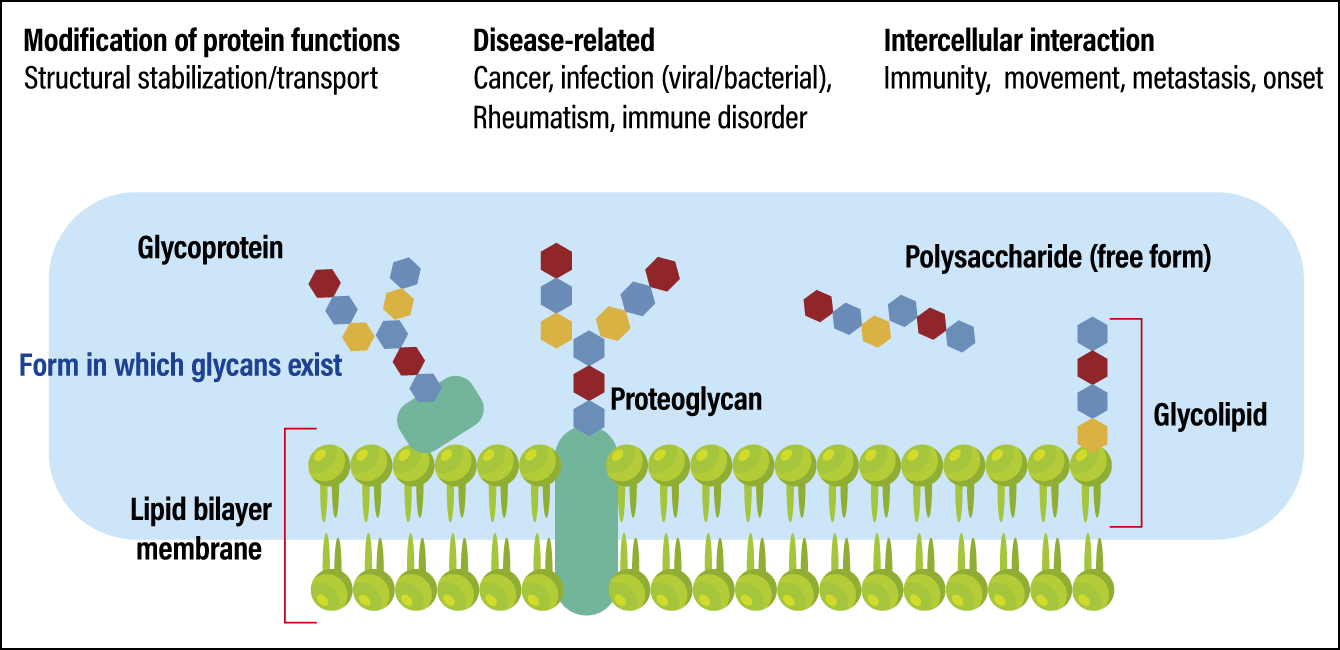
Figure 2. Functions of diverse glycans in a cellWhen a glycan attaches itself to a cell membrane protein or enters an antibody, it exists in a form that is more or less linked to the protein or lipid and carries out tuning functions within the body that are associated with the modification of protein functions, disease, and intercellular interaction.
First of all, glycans modify protein functions and identify the whereabouts of cells. The diverse proteins produced in cells are each modified by glycans and carried to the places where they ought to be. They serve as markers indicating protein functions.
Glycans also provide physical support by stabilizing protein structures. If a cell’s structure is not firmly maintained, that cell cannot carry out its functions.
Another function of glycans is determining the timing of protein metabolism. The life span of proteins in the body or blood is determined by the glycans that modify them. If just one of the glycans linked to a protein is missing, that protein could be metabolized and excreted immediately, or, conversely, have its lifespan of just a few days extended to over a week.
In the context of diseases, glycans are used as markers for recognizing what originates from the individual (self) and what does not (nonself) in such cases as cancer, rheumatism, infection, and immune disorders. It would be fair to say that it is only natural that glycans, which handle the fluctuating phenomenon of tuning the body, should be involved in work with ambiguous boundaries—namely, the recognition of self and nonself.
In the case of infections, the virus or bacterium causing the infection targets the glycans covering cell surfaces.
For example, influenza viruses target a sugar called sialic acid, which links to the end of a glycan on the surface of red blood cells (Figure 3). The virus sticks to this glycan and enters the cell, where it causes its own RNA to proliferate and emerge from the cell, leading to the onset of the disease. The avian influenza virus targets an avian glycan with an alpha-2,3 linkage.
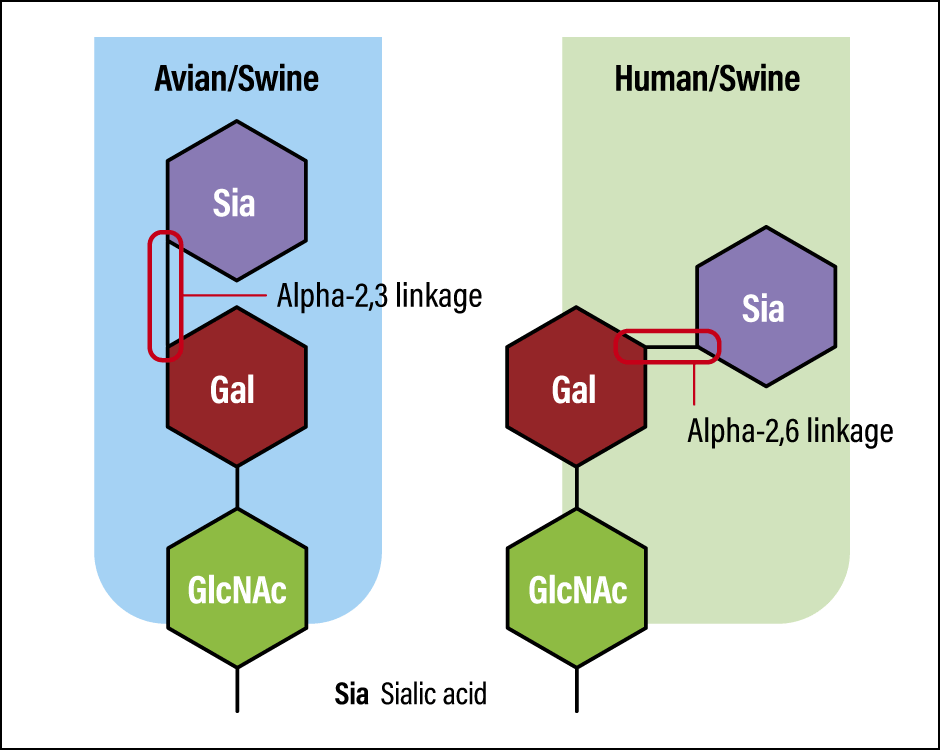
Figure 3. Influenza and glycan linkagesThe influenza virus targets sialic acid (Sia), a glycan on the surface of red blood cells. Glycans also play an important role in infection.
Produced to support evolution
Human glycans, on the other hand, have an alpha-2,6 linkage, so the avian influenza virus cannot infect humans as it is. However, the unfortunate thing is that pig glycans have both alpha-2,3 and alpha-2,6 linkages. Accordingly, the avian influenza virus can infect pigs, and if it then mutates in a pig’s body, the avian influenza virus will be able to infect humans. If this virus becomes highly virulent, it could potentially cause a pandemic similar to COVID-19.
So, why did glycans come to have such diverse functions?
Organisms produced glycans for the purpose of interaction with the outside world and between the interior and exterior of cells. In other words, it is thought that the purpose of glycans was to support evolution.
Scientists think that, in the environment on Earth in ancient times, it was possible to synthesize monosaccharides such as glucose and mannose by means of chemical reactions alone—in other words, without the need for intervention by organisms. Accordingly, these monosaccharides were already in existence when living organisms emerged, so organisms would have been able to use these sugars as an energy source. It is probably because of this long history that we primarily see sugars (monosaccharides) as a source of energy.
As a result of evolution, phytoplankton capable of photosynthesis subsequently emerged and organisms acquired a new system for obtaining energy by metabolizing lipids. Highly evolved organisms like we humans use a hybrid of these old and new metabolic systems.
Nevertheless, the core realm for organisms, namely the central nervous system, remains highly reliant on glucose as an energy source. Given that lipids are a highly efficient energy source, why do our bodies not use these substances? The answer is that, while lipids provide a great deal of energy, they are also hard to control.
Genes can acquire diversity via glycans
As stated above, glucose is a remarkably stable substance that has existed since before the emergence of living organisms. By obtaining energy from glucose, organisms have succeeded in constantly evolving ever since they first emerged. If that is the case, it means no other substance is as reliable as a source of energy for the central nervous system, which serves as the control tower for supporting life. On Earth in ancient times, the sugars that could be synthesized by the chemical reactions of organisms alone included not only glucose, but also mannose and fructose. These sugars were important sources of energy for all flora and fauna.
However, at the same time, various components were required for them to evolve into more complex, advanced organisms. The table shows the sizes of the whole genomes of a number of species and the number of genes in each. In terms of body size, Escherichia coli (E. coli ), for example, is 2-4 μm, so a human around 160 cm tall is about 400,000-800,000 times larger, but the size of the E. coli genome is 4.64 Mbp, while a human’s is just 645 times larger, at approximately 3,000 Mbp. The difference between a fruit fly (Drosophila) and a human is only 25 times.
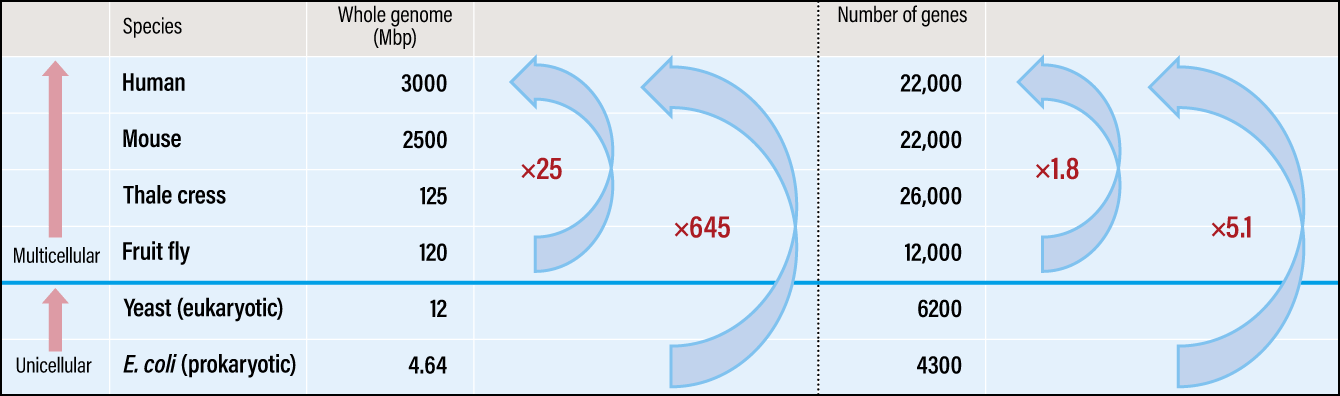
Table. Genome size and number of genes by speciesOne can see that, based on genes and nucleic acids alone, there are not enough components to evolve into a complex species like humans.
Next, within the genome, let us compare the number of genes, which code proteins. The number of genes in humans and mice hardly differs at all. The difference between fruit flies and humans is 1.8 times, while the plant thale cress (Arabidopsis thaliana) actually has more genes than a human.
Let us now look at the three major life chains. Nucleic acids and amino acids have only one type of linkage system. In contrast, sugars use hydroxyl group (OH group) bonds and can generate 10 different linkages. For example, if we compare chains respectively consisting of six nucleic acid molecules, six amino acid molecules, and six sugars, the number of possible linkage variations in the case of DNA, which consists of four types of nucleic acid (A.T.G.C), is 4 to the power of 6, while there are around 20 types of amino acid, so the number of possible peptides (proteins) is 20 to the power of 6. In contrast, the types of glycans to which the various types of sugar can link actually number in excess of 1 trillion. In other words, genes and nucleic acids alone lack sufficient components to produce diversity. It would be fair to say that it is sugars, in the form of glycans, that have fully filled this gap.
It may well be that organisms initially made small changes to glucose and other monosaccharides in order to use them, but as they evolved, these organisms produced enzymes, enabling them to alter the original sugars, produce new and different sugars, and link them together. Once polysaccharides were created via the intervention of organisms in this way, the uses of sugars steadily expanded as organisms began to use them as the support medium for their cells or as energy storage. Glycans could be described as the final evolved form of these sugars (Figure 4).
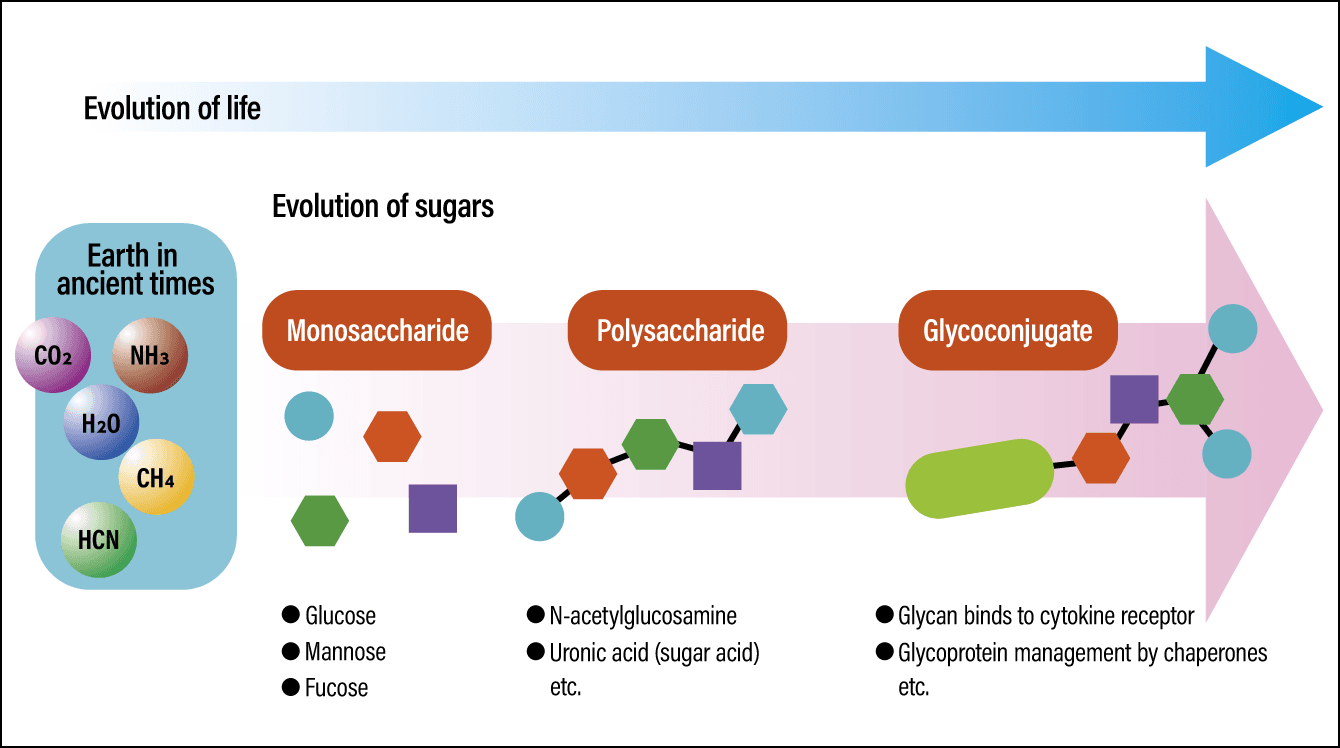
Figure 4. Sugars essential to the evolution of lifeScientists believe that early organisms on Earth in ancient times synthesized new sugars by means of linking other sugars to glucose, such as N-acetylglucosamine (GlcNAc), which is more or less a modified form of glucose. Once this was not enough to provide all the components required, organisms began using mannose. Then, pursuing further diversity, they began to produce polysaccharides from sugars such as galactose and fucose, before creating more complex glycans. As galactose cannot be produced without the intervention of an organism, the link between the evolution of sugars and glycans and biological evolution can be seen. In most cases, glycans exist alongside proteins and lipids, so they formed links to various molecules and began to regulate their functions.
As stated earlier, glycans are present in the body in forms bonded to proteins and lipids; in fact, hardly any glucose at all is used in the component sugars of the N-glycans linked to asparagine. This is because organisms use glucose as a valuable source of energy. In using sugars, organisms maintain a strict distinction between sugars as an energy source, and sugars and glycans as biochemical substances. The evolution from glucose into various sugars is the work of life itself, continuing in an unbroken process.
In our studies of plant glycans, we use microalgae in the form of red tide plankton. These plankton are unicellular organisms that combine the features of animals and plants, as they are motile and also carry out photosynthesis, and therefore are positioned at the base of the phylogenetic tree of flora and fauna. The N-glycans in these plankton are almost exclusively mannose. N-glycans in an early stage bonded first with N-acetylglucosamine (GlcNAc), which could be described as a modified form of glucose, and then later with mannose, but they did not attach other sugars. Once these evolved into mosses and ferns, xylose and other sugars began to be added.
Furthermore, once these evolved into such advanced forms as angiosperms and gymnosperms, complex systems using a variety of information were required in order to produce stems, trunks and fruit. Accordingly, a wider variety of sugars began to be used as components to produce diversity. In fact, major events caused the evolution from mosses into cypresses. It has become apparent that, when this occurred, glycans also changed at the same time.
We are currently analyzing the glycans of cereals, with the aim of comprehensively analyzing the relation between glycans and plant evolution, and putting our findings to practical use.
As the three major cereals rice, wheat, and corn have repeatedly undergone selective breeding to improve yield and alter their flavor, it goes without saying that there are substantial differences between the glycans in the improved varieties and those in the wild types. The wild types would likely have amassed glycans enabling them to adapt to changes in harsh environments, whereas improved cereals designed to be raised in hothouses with a regulated environment for the purpose of maximizing the quantity harvested are vulnerable to environmental changes. When subjected to changes in the environment, the orderly arrangement of glycans in the improved varieties is disrupted. We are conducting structural analysis of these glycans to investigate what differences in glycans are caused by the environment. Our hope is that this work might make it possible to overcome the vulnerabilities of the improved varieties and restore their resilience.
Organisms created glycans by selecting appropriate sugars, in order to adapt to environmental changes. Understanding glycans requires us to understand the evolution of sugars. This in turn will help us to shed light on the evolution of living organisms.